|
Post by Admin on Mar 31, 2020 21:15:14 GMT
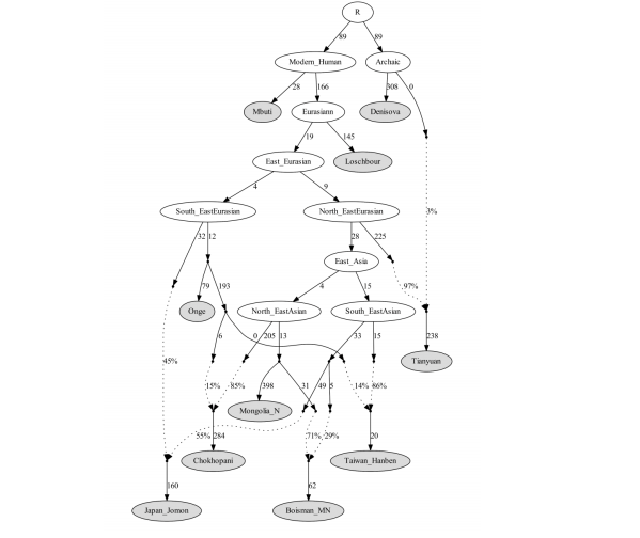 Figure 5: qpGraph modeling of a subset of East Asians. We used all available sites in the 1240K dataset, restricting to transversions only to replicate key results (Supplementary Information). We started with a skeleton tree that fits the data with Denisova, Mbuti, Onge, Tianyuan and Loschbour and one admixture event. We then grafted on Mongolia_East_N, Jomon, Taiwan_Hanben, Chokhopani, and Boisman in turn, adding them consecutively to all possible edges in the tree and retaining only graph solutions that provided no differences of |Z|>3 between fitted and estimated statistics. We used the MSMC relative population split time to constrain models (the maximum discrepancy for this model is |Z|=2.8). Drifts along edges are multiplied by 1000. Dashed lines represent admixture. Deep population splits are not well constrained due to a lack of data from Upper Paleolithic East Asians. 445 To obtain insight into the formation of present-day Japanese archipelago populations, 446 we searched for groups that contribute most strongly to present-day Japanese through 447 admixture f3-statistics. The most strongly negative signals come from mixtures of Han 448 Chinese and ancient Jomon (f3(Japanese; Han Chinese, Jomon)) (Table S16). We can 449 model present-day Japanese as two-way mixtures of 84.3% Han Chinese and 15.7% 450 Jomon or 87.6% Korean and 12.4% Jomon (we cannot distinguish statistically 451 between these two sources; Table S17 and Table S18). This analysis by no means 452 suggests that the mainland ancestry in Japan was contributed directly by the Han 453 Chinese or Koreans themselves, but does suggest that it is from an ancestral 454 population related to those that contributed in large proportion to Han Chinese as well 455 as to Koreans for which we do not yet have ancient DNA data. 456 457 We used qpGraph35 to explore models with population splits and gene flow, and 458 tested their fit to the data by computing f2-, f3- and f4- statistics measuring allele 459 sharing among pairs, triples, and quadruples of populations, evaluating fit based on 460 the maximum |Z|-score comparing predicted and observed values. We further 461 constrained the models by using estimates of the relative population split times 462 between the selected pairs of populations based on the output of the MSMC 463 software50. While admixture graph modeling based on allele frequency correlation 464 statistics is not able to reject a model in which ancient Taiwan individuals and 465 Boisman share substantial ancestry with each other more recently than either does 466 with the ancestors of Chokopani and Core Tibetans, this model cannot be correct 467 because our MSMC analysis reveals that Core Tibetans (closely related to Chokopani) 468 and Ulchi (closely related to Boisman) share ancestry more recently in time on 469 average than either does with Ami (related to Taiwan_Hanben). This MSMC-based 470 constraint allowed us to identify a parsimonious working model for the deep history 471 of key lineages discussed in this study (Supplementary Information section 3: 472 qpGraph Modeling). Our fitted model (Figure 5), suggests that much of East Asian 473 ancestry today can be modelled as derived from two ancient populations: one from the 474 same lineage as the approximately ~40,000-year-old Tianyuan individual and the 475 other more closely related to Onge, with groups today having variable proportions of 476 ancestry from these two deep sources. In this model, the Mongolia_East_N and Amur 477 River Basin Boisman related lineages derive the largest proportion of their ancestry 478 from the Tianyuan-related lineage and the least proportion of ancestry from the Onge 479 related lineage compared with other East Asians. A sister lineage of 480 Mongolia_East_N is consistent with expanding into the Tibetan Plateau and mixing 481 with the local hunter-gatherers who represent an Onge-related branch in the tree. The 482 Taiwan Hanben are well modelled as deriving about 14% of their ancestry from a 483 lineage remotely related to Onge and the rest of their ancestry from a lineage that also 484 contributed to Jomon and Boisman on the Tianyuan side, a scenario that would 485 explain the observed affinity among Jomon, Boisman and Taiwan Hanben. We 486 estimate that Jomon individuals derived 45% of their ancestry from a deep basal 487 lineage on the Onge side. These results are consistent with the scenario a Late 488 Pleistocene coastal route of human migration linking Southeast Asia, the Japanese 489 Archipelago and the Russian Far East51. Due to the paucity of ancient genomic data 490 from Upper Paleolithic East Asians, there are limited constraints at present for 491 reconstructing the deep branching patterns of East Asian ancestral populations, and it 492 is certain that this admixture graph is an oversimplification and that additional 493 features of deep population relationships will be revealed through future work. 495 At the end of the last Ice Age, there were multiple highly differentiated populations in 496 East as well as West Eurasia, and it is now clear that these groups mixed in both 497 regions, instead of one population displacing the others. In West Eurasia, there were 498 at least four divergent populations each as genetically differentiated from each other 499 as Europeans and East Asians today (average FST=0.10), which mixed in the 500 Neolithic, reducing heterogeneity (average FST=0.03) and mixed further in the Bronze 501 Age and Iron Age to produce the present-relatively low differentiation that 502 characterizes modern West Eurasia (average FST=0.01)52. In East Eurasia, our study 503 suggests an analogous process, with the differentiation characteristic of the Amur 504 River Basin groups, Neolithic Yellow River farmers, and people related to those of 505 the Taiwan Iron Age (average FST=0.06 in our data) collapsing through mixture to 506 today’s relatively low differentiation (average FST=0.01-0.02) (Figure 6). A priority 507 should be to obtain ancient DNA data for the hypothesized Yangtze River population 508 (the putative source for the ancestry prevalent in the Southeast Asian Cluster of 509 present-day groups), which should, in turn, make it possible to test and further extend 510 these models, and in particular to understand if dispersals of people in Southeast Asia 511 do or do not correlate to ancient movements of people.
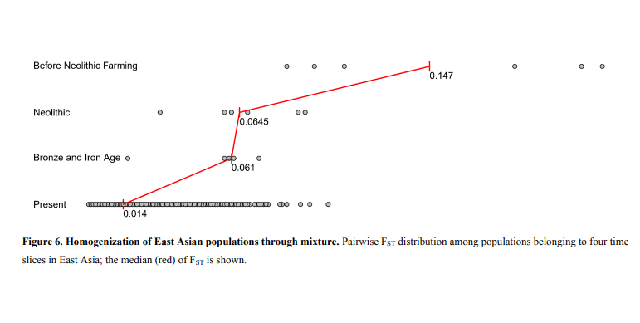
|
|
|
Post by Admin on Apr 2, 2020 5:50:36 GMT
A dynamic 6,000-year genetic history of Eurasia’s Eastern Steppe Choongwon Jeong1,2,†,*, Ke Wang1,†, Shevan Wilkin3, William Timothy Treal Taylor3,4 61 Summary 62 63 The Eastern Eurasian Steppe was home to historic empires of nomadic pastoralists, including 64 the Xiongnu and the Mongols. However, little is known about the region’s population history. 65 Here we reveal its dynamic genetic history by analyzing new genome-wide data for 214 66 ancient individuals spanning 6,000 years. We identify a pastoralist expansion into Mongolia 67 ca. 3000 BCE, and by the Late Bronze Age, Mongolian populations were biogeographically 68 structured into three distinct groups, all practicing dairy pastoralism regardless of ancestry. 69 The Xiongnu emerged from the mixing of these populations and those from surrounding 70 regions. By comparison, the Mongols exhibit much higher Eastern Eurasian ancestry, 71 resembling present-day Mongolic-speaking populations. Our results illuminate the complex 72 interplay between genetic, sociopolitical, and cultural changes on the Eastern Steppe. 73 74 Keywords 75 76 Human population history, ancient DNA, migration, nomadic pastoralists, Eastern Steppe, 77 Mongolia, Xiongnu Empire, Mongol Empire
79 Introduction 80 81 Recent paleogenomic studies have revealed a dynamic population history on the Eurasian 82 Steppe, with continental-scale migration events on the Western Steppe coinciding with Bronze 83 Age transformations of Europe, the Near East, and the Caucasus (Allentoft et al., 2015; de 84 Barros Damgaard et al., 2018; Damgaard et al., 2018; Haak et al., 2015; Mathieson et al., 2015; 85 Wang et al., 2019). However, despite advances in understanding the genetic prehistory of the 86 Western Steppe, the prehistoric population dynamics on the Eastern Steppe remain poorly 87 understood (de Barros Damgaard et al., 2018; Jeong et al., 2018). The Eastern Steppe is a great 88 expanse of grasslands, forest-steppe, and desert-steppe extending more than 2,500 km from the 89 Altai-Sayan mountain range in the west to northeastern China in the east (Fig. 1). While also 90 covering parts of modern-day China and Russia, most of the Eastern Steppe falls within the 91 national boundaries of present-day Mongolia (Fig. S1). The Eastern Steppe has been occupied 92 since the early Upper Paleolithic (ca. 34,000 cal BP) (Devièse et al., 2019), and recent 93 paleogenomic studies suggest that the eastern Eurasian forest-steppe zone was genetically 94 structured during the Pre-Bronze and Early Bronze Age periods, with a strong west-east 95 admixture cline of ancestry stretching from Botai in central Kazakhstan to Lake Baikal in 96 southern Siberia to Devil’s Gate Cave in the Russian Far East (de Barros Damgaard et al., 97 2018; Jeong et al., 2018; Sikora et al., 2019; Siska et al., 2017). 98 99 During the Bronze Age, the multi-phased introduction of pastoralism drastically changed 100 lifeways and subsistence on the Eastern Steppe (Honeychurch, 2015; Kindstedt and Ser-Od, 101 2019). A recent large-scale paleoproteomic study has confirmed milk consumption in 102 Mongolia prior to 2500 BC by individuals affiliated with the Afanasievo (3000 BCE) and 103 Chemurchek (2750-1900 BCE) cultures (Wilkin et al., 2019). Although Afanasievo groups in 104 the Upper Yenisei region have been genetically linked to the Yamnaya culture of the Pontic 105 Caspian steppe (ca. 3300-2200 BCE) (Allentoft et al., 2015; Morgunova and Khokhlova, 106 2013), the origins of the Chemurchek have been controversial (Kovalev, 2014). Once 107 introduced, ruminant dairying became widespread by the Middle/Late Bronze Age (MLBA, 108 here defined as 1900-900 BCE), being practiced in the west and north at sites associated with 109 Deer Stone-Khirigsuur Complex (DSKC) and in the east in association with the Ulaanzuukh 110 culture (Jeong et al., 2018; Wilkin et al., 2019). The relationships between DSKC and 111 Ulaanzuukh groups are poorly understood, and little is known about other MLBA burial 112 traditions in Mongolia, such as the Mönkhkhairkhan and Baitag (See supplementary materials). 113 By the end of the second millennium BCE, the previously powerful MLBA cultures were in 114 decline, and political power shifted during the Early Iron Age to the Slab Grave culture (ca. 115 1000-300 BCE), whose burials often incorporate uprooted materials from DSKC monuments 116 (Honeychurch, 2015), and to the Uyuk culture (ca. 700-200 BCE) of the Sayan mountains to 117 the northwest (also known as the Aldy-Bel culture), who had strong cultural ties to the Pazyryk 118 (ca. 500-200 BCE) and Saka (ca. 900-200 BCE) cultures of the Altai and eastern Kazakhstan 119 (Savinov, 2002; Tseveendorj, 2007).
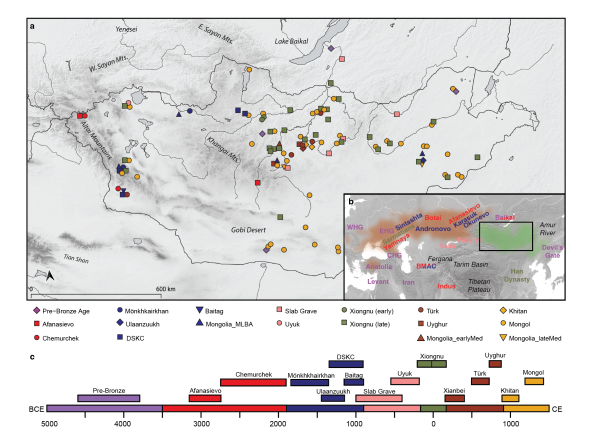 Fig. 1. Overview of ancient populations and time periods 121 From the late first millennium BC onwards, a series of hierarchical and centrally organized 122 empires arose on the Eastern Steppe, notably the Xiongnu (209 BCE-98 CE), Türkic (552-742 123 CE), Uyghur (744-840 CE), and Khitan (916-1125 CE) empires. The Mongol empire, emerging in the 13th 124 century CE, was the last and most expansive of these regimes, eventually controlling 125 vast territories and trade routes stretching from China to the Mediterranean. However, due to a 126 lack of large-scale genetic studies, the origins and relationships of the people who formed these 127 states, including both the ruling elites and local commoners, remain obscure (See 128 supplementary materials).
130 To clarify the population dynamics on the Eastern Steppe since prehistory, we generated and 131 analyzed genome-wide genetic datasets for 214 individuals from 85 Mongolian and 3 Russian 132 sites spanning approximately 6,000 years of time (ca. 4600 BCE to 1400 CE) (Tables S1-S9 133 and Fig. S2-S8) (See supplementary materials). To this, we added recently published genomic 134 data for 18 Bronze Age individuals from northern Mongolia (Jeong et al., 2018), as well as 135 datasets from neighboring ancient populations in Russia and Kazakhstan (de Barros Damgaard 136 et al., 2018; Damgaard et al., 2018; Narasimhan et al., 2019; Sikora et al., 2019; Unterländer 137 et al., 2017) (Table S10), which we analyze together with worldwide modern reference 138 populations (Table S11; Fig. S4). We also generated 24 new accelerator mass spectrometry 139 dates (Table S12), supplementing 81 previously published radiocarbon dates from Mongolia 140 (Jeong et al., 2018; Taylor et al., 2019), for a total of 105 directly dated individuals in this study 141 (See supplementary materials).
|
|
|
Post by Admin on Apr 3, 2020 6:42:51 GMT
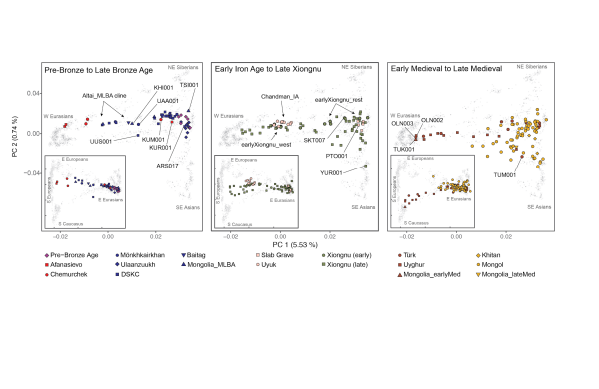 Fig. 2. Genetic structure of Mongolia through time 143 Results 144 145 Pre-Bronze Age population structure and the arrival of pastoralism 146 147 Throughout the mid-Holocene and before the Bronze Age, the Eastern Steppe was sparsely 148 populated by hunter-gatherers, whose lives and activities are recorded in petroglyphs 149 throughout the region (Jacobson-Tepfer and Meacham, 2010; Kindstedt and Ser-Od, 2019). In 150 this study, we analyzed six pre-Bronze Age individuals from three sites dating to the fifth and 151 fourth millennia BCE: one from eastern Mongolia (SOU001, “eastMongolia_preBA”, 4686- 152 4495 cal. BCE), one from central Mongolia (ERM003, “centralMongolia_preBA”, 3781-3643 153 cal. BCE), and four from the eastern Baikal region (“Fofonovo_EN”). By comparing these 154 genomes to previously published ancient and modern data across Eurasia (Fig. 2) (see Methods 155 and Materials), we found that they are most closely related to contemporaneous hunter 156 gatherers from the western Baikal region (“Baikal_EN”, 5200-4200 BCE) and the Russian Far 157 East (“DevilsCave_N”, ca. 5700 BCE), filling in the geographic gap in the distribution of this 158 genetic profile (Fig. 3a). We refer to this profile as “Ancient Northeast Asian” (ANA) to reflect 159 its geographic distribution relative to another widespread mid-Holocene genetic profile known 160 as “Ancient North Eurasian” (ANE), which is found among the Pleistocene hunter-gatherers 161 of the Mal’ta (ca. 24500-24100 BP) and Afontova Gora (ca. 16900-16500 BP) sites in Siberia 162 (Fu et al., 2016; Raghavan et al., 2015) and the horse-herders of Botai, Kazakhstan (ca. 3500- 163 3300 BCE) (de Barros Damgaard et al., 2018). In PCA (Fig. 2), ancient ANA individuals fall 164 close to the cluster of present-day Tungusic- and Nivkh-speaking populations from the lower 165 Amur River region in northeast Asia, indicating that their genetic profile is still present in 166 indigenous populations of the Far East today (Fig. S5). EastMongolia_preBA is genetically 167 indistinguishable from DevilsCave_N (Fig. 3a; Table S13; Fig. S9-S10), whereas 168 Fofonovo_EN and the slightly later centralMongolia_preBA both derive a minority (12-17%) 169 of their ancestry from ANE-related (Botai-like) groups with the remainder of their ancestry 170 (83-87%) characterized as ANA (Fig. 3a; Table S13). Reanalyzing published data from the 171 western Baikal early Neolithic Kitoi culture (Baikal_EN) and the early Bronze Glazkovo 172 culture (Baikal_EBA) (de Barros Damgaard et al., 2018), we find that they have similar 173 ancestry profiles and a slight increase in ANE ancestry through time (from 6.4% to 20.1%) 174 (Fig. 3a). Overall, these data suggest broader genetic shifts in hunter-gatherer ancestry at this 175 time. 176 177 Pastoralism in Mongolia is often assumed to have been introduced by the eastward expansion 178 of Western Steppe cultures (e.g., Afanasievo) via either the Upper Yenisei and Sayan mountain 179 region to the northwest of Mongolia or through the Altai mountains in the west (Janz et al., 180 2017). Although the vast majority of Afanasievo burials found to date are located in the Altai 181 mountains and Upper Yenisei regions outside of Mongolia (Honeychurch, 2017), an Early 182 Bronze Age (EBA) site in the southern Khangai Mountains of central Mongolia has yielded 183 Afanasievo-style graves with proteomic evidence of ruminant milk consumption (Wilkin et al., 184 2019). Analyzing two of these individuals (Afanasievo_Mongolia, 3112-2917 cal. BCE), we 185 find that their genetic profiles are indistinguishable from that of published Afanasievo 186 individuals from the Yenisei region (Allentoft et al., 2015; Narasimhan et al., 2019) (Fig. 2, 187 Fig. S11), and thus these two Afanasievo individuals confirm that the EBA expansion of 188 Western Steppe herders (WSH) did not stop at the mountain ranges separating the Western and 189 Eastern Steppes (Allentoft et al., 2015), but rather extended a further 1500 km eastwards into 190 the heart of central Mongolia (Fig. 3a). 192 Although the Afanasievo-related expansion may have introduced dairy pastoralism into 193 Mongolia (Janz et al., 2017), the people themselves do not seem to have left a long-lasting 194 genetic footprint. An Afanasievo-style burial (Afanasievo_KUR001) excavated in the 195 Mongolian Altai and dating to approximately 500 years later (2618-2487 cal. BCE), was found 196 to have a distinct (Fig. 2) and mostly ANA (~90%) derived ancestry (Fig. 3a), with only a 197 minor component tracing back to the Afanasievo (~10%; Table S14; Fig. S11).
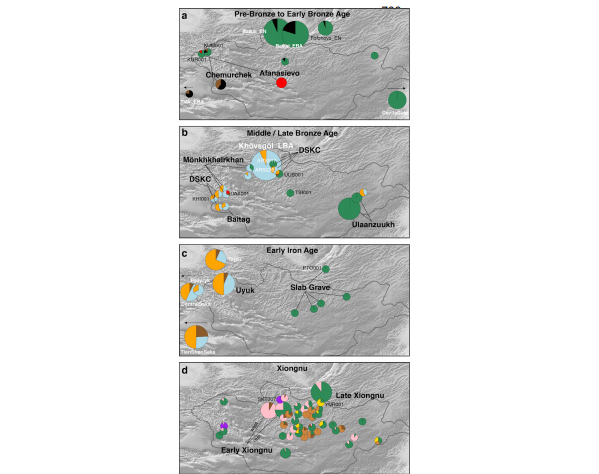 Fig. 3. Genetic changes in the Eastern Steppe across time characterized by 736 qpAdm. 198 199 The succeeding EBA Chemurchek culture (2750-1900 BCE), a ruminant dairying society 200 (Wilkin et al., 2019) whose mortuary features include stone slabs and anthropomorphic stelae, 201 has also been purportedly linked to WSH migrations (Kovalev and Erdenebaatar, 2009). 202 Chemurchek graves are found throughout the Altai and in the Dzungar Basin in Xinjiang, China 203 (Jia and Betts, 2010). We analyzed two Chemurchek individuals from the southern Altai site 204 of Yagshiin Huduu and one from Khundii Gobi (KUM001) in the northern Altai. Compared to 205 Afanasievo_Mongolia, the Yagshiin Huduu individuals also show a high degree of Western 206 ancestry but are displaced in PCA (Fig. 2), having also a strong genetic affinity with ANE207 related ancient individuals such as AfontovaGora3 (AG3), West_Siberia_N, and Botai (Fig. 208 3a; Fig. S9, S11). We find that these Chemurchek individuals (Chemurchek_Altai) are 209 genetically similar to Dali_EBA (Fig. 3a), a contemporaneous individual from eastern 210 Kazakhstan (Narasimhan et al., 2019). The genetic profiles of both the Yagshiin Huduu and 211 Dali_EBA individuals are well fitted by two-way admixture models with Botai (60-78%) and 212 groups with ancient Iranian-related ancestry, such as Gonur1_BA from Gonur Tepe, a key EBA 213 site of the Bactria-Margiana Archaeological Complex (BMAC) (22-40%; Table S15; Fig. 3a), 214 and ancient individuals from post-BMAC Bronze Age sites in southeastern Uzbekistan (Table 215 S15). Although minor genetic contributions from the Afanasievo-related groups cannot be 216 excluded, Iranian-related ancestry is required for all fitting models, and this admixture is 217 estimated to have occurred 12±6 generations earlier (~336±168 years; Fig. S12) when modeled 218 using DATES (Narasimhan et al., 2019). Because all proxy source populations used in this 219 modeling are quite distant in either time or space from the EBA Altai, the proximate 220 populations contributing to the Chemurchek cannot be precisely identified, but future research 221 in genetically unsampled regions such as Xinjiang may provide important clues. 222 223 In the northern Altai, the third Chemurchek individual in our study (KUM001) has additional 224 northeast Asian ancestry, similar to levels seen in Afanasievo_KUR001 (Fig. 3a). KUM001 225 can be modeled as a mixture of Chemurchek-related (~30%) and ANA ancestry (~70%) (Table 226 S15). The high proportions of local ANA ancestry in KUR001 and KUM001 suggest that the 227 arrival of Afanasievo and later Chemurchek pastoralists did not necessarily displace local Altai 228 peoples, despite the strong cultural and economic impact of these herders. Indeed, the cultural 229 features that they first introduced, such as mortuary mound building and dairy pastoralism, 230 continue to the present day. However, unlike in Europe, where migrating EBA steppe herders 231 had a transformative and lasting genetic impact on local populations (Allentoft et al., 2015; 232 Haak et al., 2015; Mathieson et al., 2018), we find that neither Afanasievo nor Chemurchek 233 groups left significant or enduring genetic traces into the subsequent Middle and Late Bronze 234 Ages (MLBA).
236 Bronze Age emergence of a tripartite genetic structure 237 238 Previously, we reported a shared genetic profile among EBA western Baikal hunter-gatherers 239 (Baikal_EBA) and Late Bronze Age (LBA) pastoralists in northern Mongolia 240 (Khövsgöl_LBA) (Jeong et al., 2018). This genetic profile, composed of major and minor ANA 241 and ANE ancestry components, respectively, is also shared with the earlier eastern Baikal 242 (Fofonovo_EN) and Mongolian (centralMongolia_preBA) groups analyzed in this study (Fig. 243 3a,b), suggesting a regional persistence of this genetic profile for nearly three millennia. 244 Centered in northern Mongolia, this genetic profile is distinct from that of other Bronze Age 245 groups. Overall, we find three distinct and geographically structured gene pools in LBA 246 Mongolia, with the Khövsgöl_LBA population representing one of them (Fig. 3b). The other 247 two, which we refer to as “Altai_MLBA” and “Ulaanzuuk_SlabGrave”, are described below. 248 249 During the MLBA (1900-900 BCE), as grasslands expanded in response to climate change, 250 new pastoralist cultures expanded out of inner-montane regions and across the Eastern Steppe 251 (Kindstedt and Ser-Od, 2019). This period is also notable for the first regional evidence horse 252 milking (ca. 1200 BCE; (Wilkin et al., 2019)), and a dramatic intensification of horse use, 253 including the emergence of mounted horseback riding that would have substantially extended 254 the accessibility of remote regions of the steppe. Today, horse milking is exclusively associated 255 with alcohol production (Bat-Oyun et al., 2015), and this first appearance of horse milk may 256 mark the origin of this social tradition on the Eastern Steppe. In the Altai-Sayan region, dairy 257 pastoralists associated with the Mönkhkhairkhan, Deer Stone-Khirigsuur Complex (DSKC), 258 and Baitag cultures (Altai_MLBA, n=7), all show clear genetic evidence of admixture between 259 a Khövsgöl_LBA-related ancestry and a Sintashta-related WSH ancestry (Fig. 3b). Overall, 260 they form an “Altai_MLBA” cline on PCA between Western Steppe groups and the 261 Baikal_EBA/Khövsgöl_LBA cluster (Fig. 2), with their position varying on PC1 according to 262 their level of Western ancestry (Table S16). 263 264 This is the first appearance on the Eastern Steppe of a Sintashta-like ancestry (frequently 265 referred to as “steppe_MLBA” in previous studies), which is distinct from prior Western 266 ancestries present in the Afanasievo and Chemurchek populations, and which instead shows a 267 close affinity to European Corded-Ware populations and later Andronovo-associated groups, 268 such as the Sintashta (Allentoft et al., 2015). In Khovd province, individuals belonging to 269 Mönkhkhairkhan and DSKC cultures (SBG001 and BER002, respectively) have a similar 270 genetic profile that is best modeled as an equal mixture of Khövsgöl_LBA and Sintashta (Fig. 271 3b; Table S16). This genetic profile matches that previously described for a genetic outlier in 272 northern Mongolia that deviated from the Khövsgöl_LBA cluster in a previous study (ARS026; 273 (Jeong et al., 2018)). An additional four Altai_MLBA individuals belonging to Baitag 274 (ULI003), DSKC (ULI001), and an unclassified culture (BIL001, ULZ001) also fit this 275 admixture model with varying admixture proportions (Table S16). Taken together, the 276 Altai_MLBA cline reveals the ongoing mixture of two source populations: a 277 Sintashta/Andronovo-related WSH population and a local population represented by 278 Khövsgöl_LBA. The admixture is estimated to have occurred only 9±2 generations (~250 279 years) before the individuals analyzed in this study, a finding consistent with their 280 heterogeneous ancestry proportions (Fig. S12 and S13). Because the Sintashta culture (ca. 281 2800-1600 BCE) is associated with novel transportation technologies, such as horse-drawn 282 chariots (Anthony, 2010), the appearance of this ancestry profile on the Eastern Steppe suggests 283 that heightened mobility capabilities played an important role in linking diverse populations 284 across the Eurasian Steppe. 285 286 Three MLBA individuals in our dataset present genetic profiles that cannot be fully explained 287 by the Altai_MLBA cline. The Altai Mönkhkhairkhan individual UAA001 matches with 3- 288 way admixture models using Khövsgöl_LBA/Baikal_EBA as the eastern Eurasian source, 289 Afanasievo as the WSH source (but not Sintashta), and adding Gonur1_BA as a third source 290 (Table S16). Thus, a minor Iranian-related ancestry component is necessary to model this 291 individual’s genetic profile, as reflected by his displacement along PC2 in Fig. 2. We also find 292 that the culturally unclassified Altai individual KHI001 may need additional ancestry from 293 Gonur1_BA to improve the existing 2-way admixture model. Last, UUS001, despite coming 294 from a DSKC context in Khövsgöl province, fits better with a 3-way model of using 295 eastMongolia_preBA and Gonur1_BA with Sintashta as the WSH source. Although cultural 296 differences may have existed among the major MLBA mortuary traditions of the Altai and 297 northern Mongolia (Mönkhkhairkhan, DSKC, and Baitag), they do not form distinct genetic 298 groups. Rather, these mortuary traditions encompass individuals of disparate and recently 299 admixed ancestry, and the proliferation and variability of mortuary features at this time likely 300 reflects this demographic dynamism. 302 The populations making up the heterogeneous Altai_MLBA cline left descendants in the Altai303 Sayan region, who we later identify at the Uyuk site of Chandman Mountain (“Chandman_IA”, 304 ca. 400-200 BCE) in northwestern Mongolia during the Early Iron Age (EIA). Nine 305 Chandman_IA individuals form a tight cluster on PCA at the end of the previous Altai_MLBA 306 cline away from Khövsgöl_LBA cluster (Fig. 2). During the EIA, the Uyuk (also known as the 307 Aldy-Bel or Sagly-Bazhy culture) were pastoralists largely centered in the Upper Yenisei 308 region of present-day Tuva, and together with the Pazyryk, with whom they share log chamber 309 tombs and other features, they formed part of a broader Saka cultural phenomenon that 310 stretched across the Western Steppe, the Tarim Basin, and the Upper Yenisei (Parzinger, 2006). 311 In addition to dairying, many Saka groups, including EIA individuals at the Chandman 312 Mountain site, also cultivated millet (Murphy et al., 2013; Ventresca Miller and Makarewicz, 313 2019; Wilkin et al.), a practice that had been earlier adopted by many southern steppe and 314 Central Asian populations during the westward spread of millet from China to the Caucasus 315 during the second millennium BCE (Ventresca Miller and Makarewicz, 2019). 316 317 We find that EIA Saka populations systematically deviate from the earlier Altai_MLBA cline, 318 requiring a third ancestral component (Fig. 3c). The appearance of this ancestry, related to 319 populations of Central Asia (Caucasus/Iranian Plateau/Transoxiana regions) including BMAC 320 (Narasimhan et al., 2019), is clearly detected in the Iron Age groups such as Central Saka 321 (Damgaard et al., 2018), TianShan Saka (Damgaard et al., 2018), Tagar (Damgaard et al., 322 2018), and Chandman_IA (Tables S17-S18), while absent in the earlier DSKC and Karasuk 323 groups (Tables S16-S17). This third component makes up 6-24% of the ancestry in these Iron 324 Age groups, and the date of admixture in Chandman_IA is estimated ~17±4 generations earlier, 325 ca. 750 BCE, which postdates the collapse of the BMAC ca. 1600 BCE and slightly predates 326 the formation of the Persian Achaemenid empire ca. 550 BCE (Fig. S12, S13). We suggest that 327 this Iranian-related genetic influx was mediated by increased contact and mixture with 328 agropastoralist populations in the region of Transoxiana (Turan) and Fergana during the LBA 329 to EIA transition. The widespread emergence of horseback riding during the late second and 330 early first millennium BCE (Drews, 2004), and the increasing sophistication of horse transport 331 thereafter, likely contributed to increased population contact and the dissemination of this 332 Iranian-related ancestry onto the steppe. Our results do not exclude additional spheres of 333 contact, such as increased mobility along Inner Asian Mountain Corridor, which could have 334 also introduced this ancestry into the Altai via Xinjiang starting in the Bronze Age (Frachetti, 335 2012). 336 337 In contrast to the MLBA and EIA cultures of the Altai and northern Mongolia, different burial 338 traditions are found in the eastern and southern regions of Mongolia (Honeychurch, 2015), 339 notably the LBA Ulaanzuukh (1450-1150 BCE) and EIA Slab Grave (1000-300 BCE) cultures. 340 In contrast to other contemporaneous Eastern Steppe populations, we find that individuals 341 associated with these burial types show a clear northeastern-Eurasian (ANA-related) genetic 342 profile lacking both ANE and WSH admixture (Fig. 2; Fig. 3c; Fig. S7). Both groups were 343 ruminant pastoralists, and the EIA Slab Grave culture also milked horses (Wilkin et al., 2019). 344 The genetic profiles of Ulaanzuukh and Slab Grave individuals are genetically 345 indistinguishable (Fig. 2 and Table S16), consistent with the archaeological hypothesis that the 346 Slab Grave tradition emerged out of the LBA Ulaanzuukh (Honeychurch, 2015; Khatanbaatar, 347 2019). Both groups are also indistinguishable from the earlier eastMongolia_preBA individual 348 dating to ca. 4600 BCE, suggesting a long-term (>4,000 year) stability of this prehistoric 349 eastern Mongolian gene pool (Table S16). In subsequent analyses, we merged Ulaanzuukh and 350 Slab Grave into a single genetic group (“Ulaanzuukh_SlabGrave”). The 351 Ulaanzuukh_SlabGrave genetic cluster is the likely source of the previously described DSKC 352 eastern outlier from Khövsgöl province (ARS017) (Jeong et al., 2018), as well as a culturally 353 unclassified individual (TSI001) from central Mongolia who dates to the LBA-EIA transition 354 (Figs. 2 and 3c; Table S16). In addition, the Mönkhkhairkhan individual KHU001 from 355 northwest Mongolia has a non-negligible amount of Ulaanzuukh_SlabGrave ancestry in 356 addition to his otherwise Baikal_EBA ancestry (Fig. 3c; Table S16). While these three 357 individuals attest to occasional long-distance contacts between northwestern and eastern 358 Mongolia during the LBA, we find no evidence of Ulaanzuukh_SlabGrave ancestry in the 359 Altai, and the overall frequency of the Ulaanzuukh_SlabGrave genetic profile outside of 360 eastern and southern Mongolia during the MLBA is very low. During the EIA, the Slab Grave 361 culture expanded northwards, often disrupting and uprooting former DSKC graves in their path 362 (Honeychurch, 2015), and it ultimately reached as far north as the eastern Baikal region, which 363 is reflected in the genetic profile of the Slab Grave individual PTO001 in this study (Fig. 3c). 364 Overall, our findings reveal a strong east-west genetic division among Bronze Age Eastern 365 Steppe populations through the end of the Early Iron Age.
|
|
|
Post by Admin on Apr 4, 2020 5:41:34 GMT
367 The Xiongnu Empire, the rise of the first imperial steppe polity 368 369 Arising from the prehistoric populations of the Eastern Steppe, large-scale polities began to 370 develop during the late first millennium BCE. The Xiongnu was the first historically 371 documented empire founded by pastoralists, and its establishment is considered a watershed 372 event in the sociopolitical history of the Eastern Steppe (Brosseder and Miller, 2011; 373 Honeychurch, 2015). The Xiongnu held political dominance in East and Central Asia from the 374 third century BCE through the first century CE. The cultural, linguistic and genetic make-up 375 of the people who constituted the Xiongnu empire has been of great academic interest, as has 376 their relationship to other contemporaneous and subsequent nomadic groups on the Eastern 377 Steppe. Here we report genome-wide data for 60 Xiongnu-era individuals from across 378 Mongolia and dating from 350 BCE to 130 CE, thus spanning the entire period of the Xiongnu 379 empire. Although most individuals date to the late Xiongnu period (after 50 BCE), 13 380 individuals predate 100 BCE and include 12 individuals from the northern early Xiongnu 381 frontier sites of Salkhityn Am (SKT) and Atsyn Gol (AST) and one individual from the early 382 Xiongnu site of Jargalantyn Am (JAG) in eastern Mongolia. 383 384 We observe two distinct demographic processes that contributed to the formation of the early 385 Xiongnu. First, half of the individuals (n=6) form a genetic cluster (earlyXiongnu_west) 386 resembling that of Chandman_IA of the preceding Uyuk culture from the Altai-Sayan region 387 (Fig. 2). They derive 91.8±1.9% of their ancestry from Chandman_IA with the remainder 388 attributed to additional Iranian-related ancestry, which we model using BMAC as a proxy (Fig. 389 3d; Table S19). This suggests that the low-level Iranian-related gene flow identified among the 390 Chandman Mountain Uyuk during the EIA likely continued during the second half of the first 391 millennium BCE, spreading across western and northern Mongolia. Second, six individuals 392 (“earlyXiongnu_rest”) fall intermediate between the earlyXiongnu_west and 393 Ulaazuukh_SlabGrave clusters; four carry varying degrees of earlyXiongnu_west (39-75%) 394 and Ulaazuukh_SlabGrave (25-61%) related ancestry, and two (SKT004, JAG001) are 395 indistinguishable from the Ulaanzuuk_SlabGrave cluster (Fig. 3d; Tables S19-S20). This 396 genetic cline linking the earlyXiongnu_west and Ulaanzuukh_SlabGrave gene pools signifies 397 the unification of two deeply diverged and distinct lineages on the Eastern Steppe - between 398 the descendants of the DSKC, Mönkhkhairkhan, Baitag, and Uyuk cultures in the west and the 399 descendants of the Ulaanzuuk and Slab Grave cultures in the east - and even tracing back before 400 the Bronze Age, when genetic differentiation is seen even among the northern/western and 401 eastern hunter-gatherers. Overall, the low-level influx of Iranian-related gene flow continuing 402 from the previous Uyuk culture and the sudden appearance of a novel east-west mixture uniting 403 the gene pools of the Eastern Steppe are the two defining demographic processes associated 404 with the rise of the Xiongnu. 405 406 Among late Xiongnu individuals, we find even higher genetic heterogeneity (Fig. 2), and their 407 distribution on PC indicates that the two demographic processes evident among the early 408 Xiongnu continued into the late Xiongnu period, but with the addition of new waves and 409 complex directions of gene flow. Of the 47 late Xiongnu individuals, half (n=26) can be 410 adequately modelled by the same admixture processes seen among the early Xiongnu: 22 as a 411 mixture of Chandman_IA+Ulaanzuuk_SlabGrave, two (NAI002, TUK002) as a mixture of 412 either Chandman_IA+BMAC or Chandman_IA+Ulaanzuuk_SlabGrave+BMAC, and two 413 (TUK003, TAK001) as a mixture of either earlyXiongnu_west+Ulaanzuukh_SlabGrave or 414 earlyXiongnu_west+Khovsgol_LBA (Fig 3d). A further two individuals (TEV002, BUR001) 415 also likely derive their ancestry from early Xiongnu gene pool, although the p-value of their 416 models is slightly lower than the 0.05 threshold (Table S20). However, a further 11 late 417 Xiongnu with the highest proportions of western Eurasian affinity along PC1 cannot be 418 modelled using BMAC or any other ancient Iranian-related population. Instead, they fall on a 419 cluster of ancient Sarmatians from various locations in the western and central steppe (Fig. 2). 420 421 Admixture modeling confirms the presence of a Sarmatian-related gene pool among the late 422 Xiongnu: three individuals (UGU010, TMI001, BUR003) are indistinguishable from 423 Sarmatian, two individuals (DUU001, BUR002) are admixed between Sarmatian and BMAC, 424 three individuals (UGU005, UGU006, BRL002) are admixed between Sarmatian and 425 Ulaanzuuk_SlabGrave, and three individuals (NAI001, BUR004, HUD001) require Sarmatian, 426 BMAC and Ulaanzuuk_SlabGrave (Fig. 3d; Table S20. In addition, eight individuals with the 427 highest eastern Eurasian affinity along PC1 are distinct from both the Ulaanzuuk_SlabGrave 428 and Khövsgöl_LBA genetic profiles, showing affinity along PC2 towards present-day people 429 from East Asia further to the south (Fig. 2). Six of these individuals (EME002, ATS001, 430 BAM001, SON001, TUH001, YUR001) are adequately modeled as a mixture of 431 Ulaanzuuk_SlabGrave and Han (Table S19-S20), and YUR001 in particular exhibits a close 432 genetic similarity to two previously published Han empire soldiers (Damgaard et al., 2018), 433 whose genetic profile we refer to as “Han_2000BP” (Table S20). The remaining two 434 individuals (BRU001, TUH002) are similar but also require the addition of Sarmatian ancestry 435 (Fig. 3d, Table S20). The late Xiongnu are thus characterized by two additional demographic 436 processes that distinguish them from the early Xiongnu: gene flow from a new Sarmatian 437 related Western ancestry source and intensified interaction and mixture with people of the 438 contemporaneous Han empire of China. Together, these results match well with historical 439 records documenting the political influence that the Xiongnu exercised over their neighbors, 440 including the Silk Road kingdoms of Central Asia and Han Dynasty China, as well as purported 441 migrations both in and out of Mongolia (Miller, 2014). Overall, the Xiongnu period can be 442 characterized as one of expansive and extensive gene flow that began by uniting the gene pools 443 of western and eastern Mongolia and ended by uniting the gene pools of western and eastern 444 Asia.
 446 Fluctuating genetic heterogeneity in the post-Xiongnu polities 447 448 After the collapse of the Xiongnu empire ca. 100 CE, a succession of nomadic pastoralist 449 regimes rose and fell over the next several centuries across the politically fragmented Eastern 450 Steppe: Xianbei (ca. 100-250 CE), Rouran (ca. 300-550 CE), Türkic (552-742 CE), and Uyghur 451 (744-840 CE). Although our sample representation for the Early Medieval period is uneven, 452 consisting of one unclassified individual dating to the Xianbei or Rouran period (TUK001), 8 453 individuals from Türkic mortuary contexts, and 13 individuals from Uyghur cemeteries, it is 454 clear that these individuals have genetic profiles that differ from the preceding Xiongnu period, 455 suggesting new sources of gene flow into Mongolia at this time that displace them along PC3 456 (Fig. 2). Individual TUK001 (250-383 cal. CE), whose burial was an intrusion into an earlier 457 Xiongnu cemetery, has the highest western Eurasian affinity. This ancestry is distinct from that 458 of the Sarmatians, and closer to ancient populations with BMAC/Iranian-related ancestry (Fig. 459 2). Among the individuals with the highest eastern Eurasian affinity, two Türkic- and one 460 Uyghur-period individual (ZAA004, ZAA002, OLN001.B) are indistinguishable from the 461 Ulaanzuuk_SlabGrave cluster. Another individual (TUM001), who was recovered from the 462 tomb ramp of an elite Türkic-era emissary of the Tang Dynasty, has a high proportion of Han463 (78.1±1.5%) (Fig. 3e) and especially Han_2000BP-related ancestry (84±1.5%) (Table S21). 464 This male, buried with two dogs, was likely a Chinese attendant sacrificed to guard the tomb 465 entrance (Ochir et al., 2013). The remaining 17 Türkic and Uyghur individuals show 466 intermediate genetic profiles (Fig. 3e). 467 468 The high genetic heterogeneity of the Early Medieval period is vividly exemplified by twelve 469 individuals from the Uyghur period cemetery of Olon Dov (OLN; Fig. 2), in the vicinity of the 470 Uyghur capital of Ordu-Baliq. Six of these individuals came from a single tomb (grave 19), of 471 whom only two are related (OLN002 and OLN003, second-degree; Table S7); the absence of 472 closer kinship ties raises questions about the function of such tombs and the social relationships 473 of those buried within them. Most Uyghur period individuals exhibit a high, but variable, 474 degree of west Eurasian ancestry - best modeled as a mixture of Alans (a historic nomadic 475 pastoral group likely descended from the Sarmatians and contemporaries of the Huns 476 (Bachrach, 1973)) and an Iranian-related (BMAC-related) ancestry - together with 477 Ulaanzuukh_SlabGrave (ANA-related) ancestry (Fig. 3e). The admixture dates estimated for 478 the ancient Türkic and Uyghur individuals in this study correspond to ca. 500 CE: 8±2 479 generations before the Türkic individuals and 12±2 generations before the Uyghur individuals 480 (represented by ZAA001 and Olon Dov individuals).
|
|
|
Post by Admin on Apr 4, 2020 21:09:14 GMT
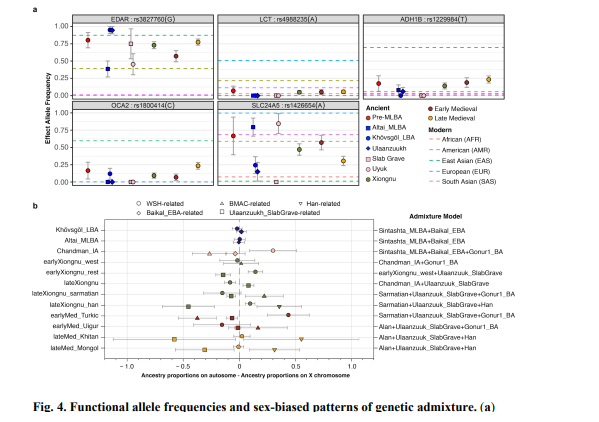 482 Rise of the Mongol empire 483 484 After the fall of the Uyghur empire in the mid-ninth century, the Khitans of northeast China 485 established the powerful Liao Dynasty in 916 CE. Although few Khitan sites are known within 486 Mongolia, the Khitans controlled large areas of the Eastern Steppe and are recorded to have 487 relocated people within their conquered territories (Kradin and Ivliev, 2008). Our study 488 includes three Khitan individuals (ZAA003, ZAA005, ULA001) from Bulgan province, all of 489 whom have a strongly eastern Eurasian (ANA and Han_2000BP-related) genetic profile (Fig. 490 2), with <10% west Eurasian ancestry (Fig. 3f; Table S22). This may reflect the northeastern 491 Asian origin of the Mongolic-speaking Khitan, but a larger sample size is required to 492 adequately characterize the genetic profile of Khitan populations within Mongolia. In 1125 CE, 493 the Khitan empire fell to the Jurchen’s Jin Dynasty, which was then conquered in turn by the 494 Mongols in 1234 CE. 495 496 At its greatest extent, the Mongol empire (1206-1368 CE) spanned nearly two thirds of the 497 Eurasian continent. It was the world’s largest contiguous land empire, and the cosmopolitan 498 entity comprised diverse populations that flowed into the steppe heartland. We analyzed 62 499 Mongol-era individuals whose burials are consistent with those of low-level, local elites. No 500 royal or regional elite burials were included, nor were individuals from the cosmopolitan 501 capital of Karakorum. Although we find that Mongol-era individuals were diverse, they exhibit 502 a much lower genetic heterogeneity compared to Xiongnu-era individuals (Fig. 2), and they 503 almost entirely lack the residual ANE-related ancestry (in the form of Chandman_IA and 504 Khövsgöl_LBA) that had been present among the Xiongnu and earlier northern/western 505 MLBA cultures. On average, Mongol period individuals have a much higher eastern Eurasian 506 affinity than previous empires, and this period marks the beginning of the formation of the 507 modern Mongolian gene pool. We find that most historic Mongols are well-fitted by a three508 way admixture model with the following ancestry proxies: Ulaanzuuk_SlabGrave, Han, and 509 Alans. Consistent with their PCA location (Fig. 2), Mongol era individuals as a group can be 510 modeled with only 15-18% of western Steppe ancestry (Alan or Sarmatian), but require 55- 511 64% of Ulaanzuuk_SlabGrave and 21-27% of Han-related ancestry (Table S22). Applying the 512 same model to each individual separately, this three-source model adequately explains 56 out 513 of 61 ancient Mongols (based on p-value at threshold of 0.05), as well as one unclassified Late 514 Medieval individual dating to around the beginning of the Mongol empire (SHU002) (Table 515 S23). 517 Since the fall of the Mongol empire in 1368 CE, the genetic profile of the Mongolian 518 populations has not substantially changed. The genetic structure established during the Mongol 519 empire continues to characterize present-day Mongolic-speaking populations living in both 520 Mongolia and Russia. We examined the genetic cladality between the historic Mongols and 521 seven present-day Mongolic-speaking groups (Mongols, Kalmyk, Buryat, Khamnegan, Daur, 522 Tu and Mongola) using an individual-based qpWave analysis {Sample; Mongolic group}. 523 Within the resolution of current data, 34/61 historic Mongols are genetically cladal with at least 524 one modern Mongolic-speaking population (Fig. S15). In addition, nearly a third of historic 525 Mongol males (12/38) have Y haplogroup C2b, which is also widespread among modern 526 Mongolians (Table S6, Fig. S3); C2b is the presumed patrilineage of Genghis Khan (Zerjal et 527 al., 2003). The Mongol empire had a profound impact on restructuring the political and genetic 528 landscape of the Eastern Steppe, and these effects endured long after the decline of the empire 529 and are still evident in Mongolia today. 530 531 Functional and gendered aspects of recurrent admixture in the Eastern Steppe 532 533 To investigate the functional aspects of recurrent admixture on the Eastern Steppe, we 534 estimated the population allele frequency of five SNPs associated with functional or 535 evolutionary aspects of lactose digestion (LCT/MCM6), dental morphology (EDAR), 536 pigmentation (OCA2, SLC24A5), and alcohol metabolism (ADH1B) (Fig. 4a). First, we find 537 that despite a pastoralist lifestyle with widespread direct evidence for milk consumption (Jeong 538 et al., 2018; Wilkin et al., 2019), the MLBA and EIA individuals of the Eastern Steppe did not 539 have any derived mutations conferring lactase persistence (LP). Individuals from subsequent 540 periods did have the derived mutation that is today widespread in Europe (rs4988235), but at 541 negligibly low frequency (~5%) and with no increase in frequency over time (Fig. 4a). This is 542 somewhat remarkable given that, in addition to other dairy products, contemporary Mongolian 543 herders consume up to 4-10 liters of airag (fermented mare’s milk, ~2.5% lactose) per day 544 during the summer months (Bat-Oyun et al., 2015), resulting in a daily intake of 100-250g of 545 lactose sugar. Petroglyph depictions of airag production date back to the EIA in the Yenisei 546 Basin (Dėvlet, 1976), and accounts of the historic Mongols record abundant and frequent 547 consumption of airag, as well as a wide range of additional liquid and solid ruminant dairy 548 products (Bayarsaikhan, 2016; Onon, 2005), which has been additionally confirmed by ancient 549 proteomic evidence (Jeong et al., 2018; Wilkin et al., 2019). How Mongolians have been able 550 to digest such large quantities of lactose for millennia in the absence of LP is unknown, but it 551 may be related to their reportedly unusual gut microbiome structure, which today is highly 552 enriched in lactose-digesting Bifidobacteria spp. (Liu et al., 2016). 553 554 Genetic markers that underwent regional selective sweeps show allele frequency changes that 555 correlate with changes in the genome-wide ancestry profile (Fig. 4a). For example, rs3827760 556 in EDAR (ectodysplasin A receptor) and rs1426654 in SLC24A5 (solute carrier family 24 557 member 5) are well-known targets of positive selection in East Asians and Western Eurasians, 558 respectively (Sabeti et al., 2007). Our MLBA and EIA populations show a strong population 559 differentiation in the allele frequencies of these two SNPs: rs3827760 frequency is much higher 560 in groups with higher eastern Eurasian affinity (Khovsgol_LBA, Ulaanzuuk_SlabGrave) while 561 rs1426654 is higher in Altai_MLBA and Chandman_IA (Table S8). We find that two SNPs 562 that have undergone more recent positive selection (Donnelly et al., 2012; Li et al., 2011) 563 among East Asians, rs1229984 in ADH1B (aldehyde dehydrogenase 1B) and rs1800414 in 564 OCA2 (oculocutaneous albinism II), were absent or in extremely low frequency during the 565 MLBA and EIA, when the eastern Eurasian ancestry was primarily ANA-related, but increased 566 in frequency over time as the proportion of East Asian ancestry increased through interactions 567 with China and other groups (Table S8). 568 569 Finally, we investigated gendered dimensions of the population history of the Eastern Steppe. 570 Sex-biased patterns of genetic admixture can be informative about gendered aspects of 571 migration, social kinship, and family structure. We observed an especially strong male-biased 572 admixture from WSH groups during the EIA and Early Medieval periods. To estimate this sex 573 biased admixture, we compared the ancestry proportions in the autosomes and X chromosome 574 for each component, and calculated the statistical significance of their difference following a 575 published strategy (Mathieson et al., 2018). When the proportion of an ancestry is higher in the 576 autosomes than in the X-chromosome, the admixture process involves male ancestors 577 harboring this ancestry in higher proportion than female ancestors. As shown in Fig. 4b, we 578 observe a clear signal of male-biased WSH admixture among the EIA Uyuk and during the 579 Türkic period (i.e., more positive Z scores), which also corresponds to the decline in the Y 580 chromosome lineage Q1a and the concomitant rise of the western Eurasian lineages such as R 581 and J (Fig. S3). During the later Khitan and Mongol empires, sex-bias Z scores shift to indicate 582 a prominant male bias for East Asian-related ancestry (Fig. S6), which can also be seen from 583 the rise in frequency of Y-chromosomal lineage O2a (Fig. S3). The Xiongnu period exhibits 584 the most complex pattern of male-biased admixture, whereby different genetic subsets of the 585 population exhibit evidence of different sources of male-biased admixture (Fig. S6). We also 586 detect ten genetic relative pairs among the Xiongnu individuals in this study, including a father587 daughter pair buried in the same grave (JAG001 and JAA001) at Jargalantyn Am, as well as a 588 mother-son pair (IMA002 and IMA005) at Il'movaya Pad, a brother-sister pair (TMI001 and 589 BUR003) at Tamiryn Ulaan Khoshuu, and a brother-brother pair (SKT002 and SKT006) at 590 Salkhityn Am (Table S7). Of the remaining six pairs, three are female-female relative pairs 591 buried within the same site, suggesting the presence of extended female kinship within 592 Xiongnu groups. These relationships, when combined with mortuary features, offer the first 593 clues to local lineage and kinship structures within the Xiongnu empire, which are otherwise 594 poorly understood.
|
|