|
Post by Admin on Jul 17, 2017 20:08:04 GMT
 Discussion The African introgression hypothesis suggests that Late Pleistocene Neanderthal mtDNAs originated through gene flow from an African source8, which we constrain taking place more than ∼270 ka (Table 1). Our analytical calculations (Supplementary Table 8 and Supplementary Note 5) show that this event is plausible even if the introgressing lineage represented a minimal proportion of the initial gene pool. This scenario reconciles the discrepancy in the nDNA and mtDNA phylogenies of archaic hominins and the inconsistency of the modern human–Neanderthal population split time estimated from nDNA and mtDNA (Fig. 1d). Under this demographical model, the Denisovan mtDNA type was common among early Neanderthals in Eurasia (for example, Sima de los Huesos) and was then largely replaced by an introgressing African mtDNA that evolved into the Late Pleistocene Neanderthal mtDNA type. While the upper bound for the time of this putative gene flow event would be the divergence time between Neanderthal and modern human mtDNAs, here dated to 413 ka (95% HPD 468–360 ka), the lower temporal limit was represented so far by the ∼160 ka TMRCA of all published Neanderthal mtDNAs (Table 1). However, the finding of the deeply diverged HST lineage splitting from the Altai branch, ∼270 ka, sets an older lower boundary for the time of this admixture event. An alternative but less parsimonious scenario is that both HST and Altai mtDNA lineages reached Eurasia independently after diverging inside Africa. In that case the suggested introgression event might have occurred later but most likely before 160 ka, our estimated date for the start of the Altai branch diversification (Fig. 1c and Table 1). The presence of modern human admixture into archaic humans has already been detected in the high coverage Neanderthal genome from the Altai region but not in sequences of chromosome 21 of two Neanderthals from Spain and Croatia14. The authors therefore suggested that a genomic contribution estimated between 0.1 and 2.1% occurred after the divergence of Altai from other late Neanderthals. However, there is a high level of uncertainty around the time of the inferred gene flow event since only one high coverage Neanderthal nuclear genome has been analysed so far. Moreover, the divergence time of the introgressing African population was estimated to date before or right after the TMRCA of modern-day humans (∼200 ka)14, while the mtDNA coalescence time between Neanderthals and modern humans is calculated at least twice as old (∼400 ka). The evolutionary scenario responsible for providing the mtDNA to the Late Pleistocene Neanderthals might have been an even earlier Middle Pleistocene gene flow from Africa, occurring in a time interval that we date between 413 and 268 ka (460–219 ka including upper and lower 95% HPD). It should be highlighted that this additional genomic contribution might have already been accounted for in ref. 14, which effectively measures the total amount of African introgression into Neanderthals after their split from Denisovans (473–381 ka; ref. 5).  The phylogenetic branch length of mtDNA sequences from 10 non-dated Neanderthal individuals was considered in BEAST, to assess individual molecular ages spanning from 130 to 40 ka. Although it is not known if the mtDNA mutation rate in modern humans is comparable to that of Neanderthals (Supplementary Note 4), molecular dating can at least be used to provide relative ages when the radiocarbon absolute chronometric method is not applicable. After the Altai mtDNA, HST is estimated to be the second oldest mtDNA with an age of 124 ka (95% HPD 183–62 ka). This wide temporal interval largely overlaps with the Marine Isotope Stage 5 (MIS 5: ∼130–73 ka)37. After its initial interglacial period (MIS 5e), central Europe was characterized by climatic fluctuations resulting in forestation cycles (MIS 5c/5a) alternated with the development of steppe-tundra biomass (MIS 5d/b)38. The stable isotopic δ13C and δ15N values of the archaic femur collagen and associated faunal remains support a more temperate, forested rather than a colder, steppe environment and is therefore consistent with an ecological context during the early warm phases of the last glaciation17. Despite having only a single complete mtDNA on the HST lineage, the two highly differentiated Neanderthal mtDNA branches suggest higher mtDNA diversity during the Middle Pleistocene, which then declined during the Late Pleistocene (Supplementary Table 4). This observation is also supported by the steady decline in mtDNA effective population size displayed in the skyline plot before a steep growth in late Neanderthal population sizes (Supplementary Fig. 7). Studies focusing on the demographic patterns of late Neanderthals who overlapped with the earliest modern humans in Europe are of key importance to understand population dynamics and interactions between archaic and modern humans. In conclusion, the HST mtDNA provided insights into the mtDNA diversity of Neanderthal populations through the Middle and Late Pleistocene. Its deep divergence time allowed us to further constrain the lower boundary for the time of the proposed African mtDNA gene flow into Neanderthal populations. The temporal corridor for this introgression event between 460 ka and 219 ka is compatible with the evidence of archaeological similarities between Africa and western Eurasia during the Lower to Middle Paleolithic transition39 and potentially may explain the dissimilarities in Middle Paleolithic industries between eastern and western Eurasia. Environmental changes across this time span might have facilitated a hominin expansion out of Africa and potentially spread cultural innovations such as the Levallois technology into Eurasia40. Alternatively, other scenarios such as multiple inventions of similar technologies by various hominin groups may explain the complex tapestry of technological variability during the late Middle Pleistocene. Nuclear data from the HST femur would be pivotal in assessing its genomic relationships with Neanderthals, Denisovans and modern humans. However, the scarce preservation of HST endogenous DNA in combination with high level of modern human contamination challenge the retrieval of its complete genome. Analyses of high-quality nDNA from more than one well-preserved Neanderthal individual are necessary to detect the consequences of African admixture into archaic human populations. Nature Communications 8, Article number: 16046 (2017)
|
|
|
Post by Admin on Jul 31, 2017 19:13:50 GMT
 Researchers at the National Institute of Mental Health (NIMH) have produced the first direct evidence that parts of our brains implicated in mental disorders may be shaped by a "residual echo" from our ancient past. The more a person's genome carries genetic vestiges of Neanderthals, the more certain parts of his or her brain and skull resemble those of humans' evolutionary cousins that went extinct 40,000 years ago, says NIMH's Karen Berman, M.D. NIMH is part of the National Institutes of Health. "It's been proposed that Neanderthals depended on visual-spatial abilities and toolmaking, for survival, more so than on the social affiliation and group activities that typify the success of modern humans -- and that Neanderthal brains evolved to preferentially support these visuospatial functions," explained Dr. Berman. "Now we have direct neuroimaging evidence that such trade-offs may still be operative in our brains." In particular, the parts of our brains that enable us to use tools and visualize and locate objects owe some of their lineage to Neanderthal-derived gene variants that are part of our genomes and affect the shape of those structures -- to the extent that an individual harbors the ancient variants. But this may involve trade-offs with our social brain. The evidence from MRI scans suggests that such Neanderthal-derived genetic variation may affect the way our brains work today -- and may hold clues to understanding deficits seen in schizophrenia and autism-related disorders, say the researchers.  The image above of MRI data shows areas of the skull preferentially affected by the amount of Neanderthal-derived DNA. During their primordial migration out of Africa, ancestors of present-day humans are thought to have interbred with Neanderthals, whose brain characteristics can be inferred from their fossilized skulls. For example, these indicate that Neanderthals had more prominent visual systems than modern humans. Might some of us, more than others, harbor Neanderthal-derived gene variants that may bias our brains toward trading sociability for visuospatial prowess -- or vice versa? The new study adds support to this possibility by showing how these gene variants influence the structure of brain regions underlying those abilities.  To test this possibility, Gregory and Berman measured the impact of Neanderthal variants on MRI measures of brain structure in a sample of 221 participants of European ancestry, drawn from the NIMH Genetic Study of Schizophrenia. The new MRI evidence points to a a gene variant shared by modern-day humans and Neanderthals that is likely involved in development of the brain's visual system. Similarly, Neanderthal variants impacting development of a particular suspect brain area may help to inform cognitive disability seen in certain brain disorders, say the researchers.
|
|
|
Post by Admin on Aug 1, 2017 19:11:50 GMT
 The most recent evolutionary relative of H. sapiens, H. neanderthalensis, represents a lineage associated with archeological samples (e.g., tools, pigments) suggesting substantial cognitive achievements1, and is typically contrasted with anatomically modern humans by particular cranial features known from fossil remains2. The initial sequencing of the Neanderthal genome summarily unearthed an unprecedented ingress to Neanderthal biology, resulting not only in a catalogue of shared ancestral alleles and divergent variation relative to H. sapiens but also evidence for gene flow from Neanderthals to modern humans3, 4, which has since been well-supported5,6,7,8. This introduction of Neanderthal alleles most likely occurred in Eurasia, as modern humans ventured out of Africa, between 47,000 and 65,000 years ago7 and accounts for about 2% of the entire non-African human genome8. The functional implications of this observation, however, are not fully understood.  If these alleles are biologically meaningful for their modern bearers5, and by extension, for their extinct originators, individuals endowed with greater proportions of Neanderthal-derived sequence differences might be expected to harbor Neanderthal-like phenotypes. To test this hypothesis, rather than using an additive model in a genetic association analysis, we performed linear regressions of polygenic measures of Neanderthal-derived common variant data with MRI imaging data. Previous reports3, 5 have described methods for testing whether single nucleotide polymorhpisms (SNPs) are derived from admixture of modern humans with Neanderthals. Here, after computing an enhanced D statistic9, which measures the percentage of these alleles present in each individual (subsequently termed “NeanderScore”), we sought to identify whether variation was associated with regional changes in skull and brain shape. Because cranial morphology is a pivotal physical element distinguishing Neanderthals from other human lineages in fossil records, it provides an ideal phenotype to test our approach and the impact of our Neanderthal-derived genetic characteristics. We anticipated that some of the Neanderthal genetic variants responsible for skull development might be represented in our sample and, when sufficiently aggregated in higher NeanderScore individuals, that these variants would be associated with hallmark Neanderthal-like morphological biases, such as increased skull length10, 11 and posterolateral broadening of the cranium2. 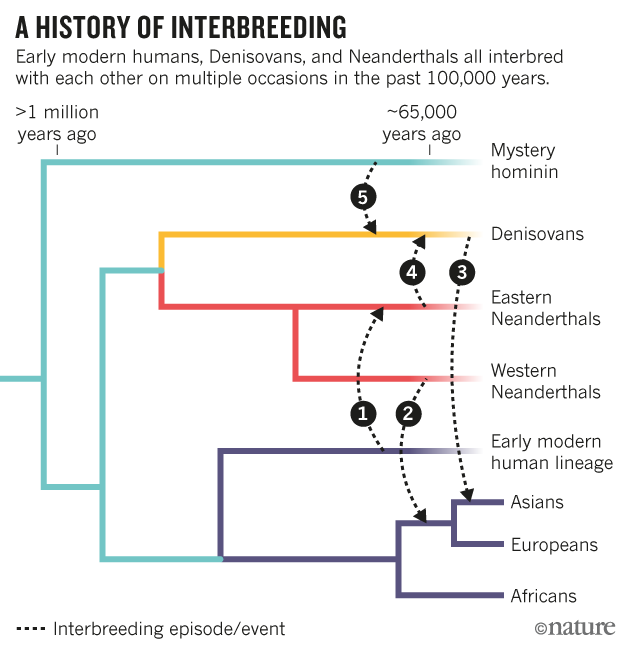 As the distinctive cranial morphology of H. neanderthalensis is hypothesized to reflect underlying brain anatomy12,13,14,15,16, in addition to characterizing the relationship between NeanderScore and modern human cranial shape, we also sought to define contributions of Neanderthal-derived genetic variation to brain structure, as measured by gray- and white-matter volume, regional sulcal depth, and gyrification index. We further hypothesized that the morphometry of brain regions underlying the identified NeanderScore-associated cranial regions would also be associated with Neanderthal genetic load.
|
|
|
Post by Admin on Aug 3, 2017 19:15:23 GMT
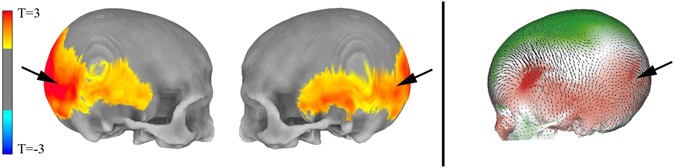 Figure 1 NeanderScore related skull shape changes. Associations between skull morphology and percentage of Neanderthal-derived SNPs (NeanderScore) in modern humans (Left), compared to differences between Neanderthal and modern human skulls (Right). Left panel shows regions with morphological changes, measured in vivo, related to NeanderScore and overlaid on the average skull of all 146 participants. Warm colors represent areas for which NeanderScore correlated with skull expansion (p < 0.05, FWE-corrected). There were no findings in the opposite direction. Right panel depicts previously published shape differences between Neanderthals and modern humans, from archeological samples, overlaid on a modern human skull. Red regions represent skull expansion in Neanderthals relative to modern humans; green represents contraction, adapted by permission from Macmillian Publishers Ltd: Nature2, © 2001. Note the convergence of in vivo and archeological data in occipito-parieto-temporal regions (arrows). In line with the contention that Neanderthal genetic load is biologically functional in modern-day humans, we first examined normalized anterior-posterior cranial length, one of the earliest reported parameters differentiating modern human and Neanderthal skulls11, and found this parameter to significantly correlate with NeanderScore (R = 0.240, p < 0.003). Examining morphometry of the entire skull in a data driven fashion, we also discovered that higher NeanderScore was positively and selectively associated with the regional size of a broad posterolateral area of the skull extending from the occipital and inferior parietal bones to bilateral temporal locales (Tmax = 3.5, p < 0.0001, family-wise error (FWE)-corrected; Fig. 1, left and middle). The area of maximal change localized to the right lambdoid suture area, involving both the occipital and parietal bones, a finding that corresponds to published shape differences between modern humans and Neanderthals as documented in fossil samples (Fig. 1, right), thus establishing the validity of our approach. To ensure the results were not driven by the population structure of the cohort, we repeated this analysis while also controlling for the first 4 dimensions from a multidimensional scaling analysis and found that the pattern of association between NeanderScore and skull shape did not significantly change from the original analysis. 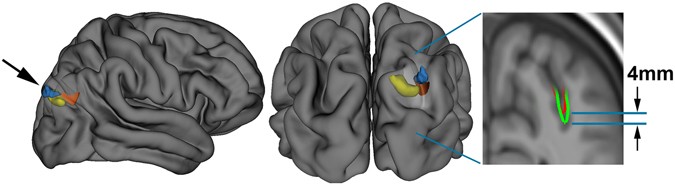 Figure 2 NeanderScore related brain changes in the intraparietal sulcus. Structural variation of the intraparietal sulcus (IPS) related to percentage of Neanderthal-derived SNPs (NeanderScore). Left and middle show lateral and posterior views of the right IPS on the average brain surface, illustrating the anatomical convergence of the associations of NeanderScore with greater sulcal depth (orange; p < 0.05 FWE-corrected), gray matter volume (blue; p < 0.005), and white matter volume (yellow; p < 0.005). Right shows a coronal slice (y = −72 mm) through the IPS where there was a sulcal depth difference of 4 mm between the 30 individuals with highest (blue line) and lowest (red line) NeanderScore. Findings are depicted on the average brain of all 221 individuals included in the study and underlie the maximal skull findings shown in Fig. 1 (arrows). Relationships between NeanderScore and Brain Morphology As previous work has related changes in skull shape to regional brain measures in European populations23, and having established that high NeanderScore is associated with statistically observable Neanderthal-like trends in modern cranial shape, we next sought to define contributions of Neanderthal-derived genetic variation to brain structure, as measured by regional sulcal depth and gyrification index, which we quantified across the entire cortical ribbon via segmentation and subsequent surface-based processing24, 25 of the full intracranial MRI dataset. We hypothesize that we might also finds similar NeanderScore-related changes in the brain regions underlying the NeanderScore-related occipito-parietal skull associations. The first measure we examined, regional sulcal depth, measures the extent to which each fold of the brain descends into the underlying mantle. We found that NeanderScore positively correlated with an increase in sulcal depth in the right intraparietal sulcus (IPS; Tmax = 4.03, pcluster < 0.001, FWE-corrected), directly beneath the area of maximal change in the prior skull shape analysis (Fig. 2), suggesting a significant but regionally circumscribed impact of Neanderthal genetic load on modern human brain.
|
|
|
Post by Admin on Aug 4, 2017 19:12:15 GMT
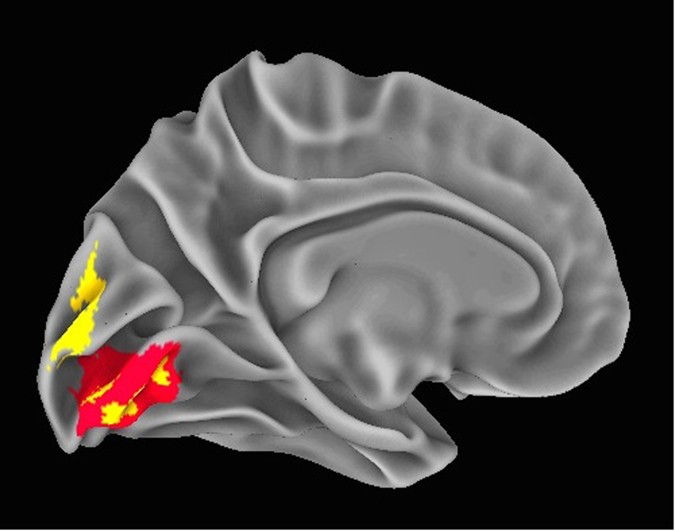 Figure 3 NeanderScore related brain changes in the primary visual cortex. Associations between local gyrification index, gray matter volume, and NeanderScore in visual cortex. Medial view of the primary visual cortex showing areas where NeanderScore was significantly associated with local gyrification index (red; p < 0.05 FWE-corrected) and with gray matter volume (yellow; p < 0.005). The statistical strength and localization of NeanderScore associations with measures of cortical folding raised the possibility of additional structural differences in these brain areas. We therefore conducted follow-up, whole-brain, voxel-based morphometric analyses aimed at quantifying associations between NeanderScore and both gray and white matter volume across the brain. Associations with both gray matter volume (MNIxyz = 28.5, −87, 36; Tmax = 3.87, p < 1.4 × 10−4, uncorrected) and white matter volume (MNIxyz = 18, −82, 28.5; Tmax = 4.32, p < 2.4 × 10−4, uncorrected) were localized to a right parietal region adjacent to the IPS focus described above, such that greater Neanderthal SNP load correlated with more gray and white matter volume (Fig. 2). Similarly, in an area overlapping with the primary visual cortex gyrification finding described above, we observed that gray matter volume, though not white matter volume, was positively associated with NeanderScore (MNIxyz = 0, −99, 10.5; Tmax = 5.07, p < 8.5 × 10−7, uncorrected; Fig. 3). We also found evidence for an inverse correlation between NeanderScore and white matter volume in the orbitofrontal cortex (MNIxyz = 37.5, 39; −7.5, Tmax = −4.44, p < 1.4 × 10−5, uncorrected), a structure that appears to have been laterally restricted in Neanderthals relative to modern H. sapiens13. 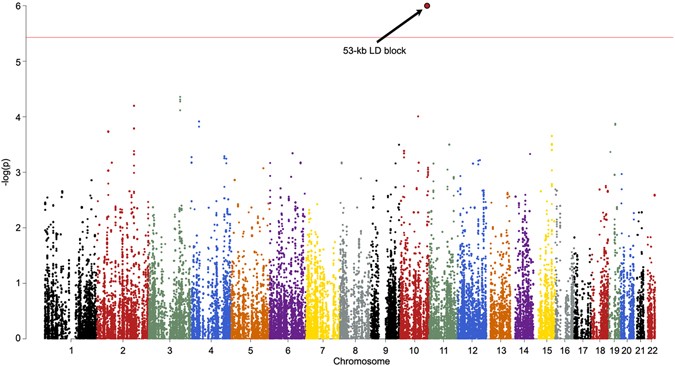 Figure 4 Manhattan plot of the shared variance of NeanderScore associated brain and skull changes. Manhattan plot showing the chromosomal position and significance of association between the shared variance of all Neanderthal-associated brain measures for each Neanderthal-derived SNP. Red line indicates Bonferroni-corrected threshold of p < 0.05 for the effective number of SNPs in the analysis. The arrow points to the significant 53-kb LD block on chromosome 10 containing 18 SNPs. See also Table 2. Post-hoc Genetic Analysis of Neanderthal-Derived SNPs Finally, we conducted an exploratory test of the association of our structural findings to specific variants within the 100,156 SNPs identified as putatively derived from Neanderthals in our participants. The convergence of findings in posterior head regions, across cranial, sulcal depth, gyrification and volumetric variables, led us to hypothesize that common genetic influences would be found in genes preferentially expressed in the human brain. Using the first component from a principal components analysis as a quantitative trait that captures the shared-variance across our structural measures, we tested each of the 100,156 Neanderthal-derived SNPs for association with brain/skull structure using a linear model. SNPs in a 53 kb LD-block of chromosome 10, encompassing the telomeric end of the gene GPR26, a G-protein coupled receptor preferentially expressed in the human brain29, showed strong association with brain/skull structure (t = 5.1, p < 1.04 × 10−6, Bonferroni-corrected p < 0.013, Table 2). Figure 4 shows the manhattan plot of these results and Fig. 5 shows the QQ plot.
|
|