|
Post by Admin on Apr 6, 2021 5:08:10 GMT
Innovative Homo sapiens behaviours 105,000years ago in a wetter Kalahari
The archaeological record of Africa provides the earliest evidence for the emergence of the complex symbolic and technological behaviours that characterize Homo sapiens1–7. The coastal setting of many archaeological sites of the Late Pleistocene epoch, and the abundant shellfish remains recovered from them, has led to a dominant narrative in which modern human origins in southern Africa are intrinsically tied to the coast and marine resources8–12, and behavioural innovations in the interior lag behind. However, stratified Late Pleistocene sites with good preservation and robust chronologies are rare in the interior of southern Africa, and the coastal hypothesis therefore remains untested. Here we show that early human innovations that are similar to those dated to around 105thousand years ago (ka) in coastal southern Africa existed at around the same time among humans who lived over 600km inland. We report evidence for the intentional collection of non-utilitarian objects (calcite crystals) and ostrich eggshell from excavations of a stratified rockshelter deposit in the southern Kalahari Basin, which we date by optically stimulated luminescence to around 105ka. Uranium–thorium dating of relict tufa deposits indicates sporadic periods of substantial volumes of fresh, flowing water; the oldest of these episodes is dated to between 110 and 100ka and is coeval with the archaeological deposit. Our results suggest that behavioural innovations among humans in the interior of southern Africa did not lag behind those of populations near the coast, and that these innovations may have developed within a wet savannah environment. Models that tie the emergence of behavioural innovations to the exploitation of coastal resources by our species may therefore require revision.
Archaeological discoveries in coastal areas of southern Africa have trans-formed our knowledge about the emergence of the complex symbolic and technological behaviours that characterize H.sapiens1–3,5,13–16. The earliest evidence for the manufacture and use of particular types of sym-bolic resources dates to about 100ka, and includes a pigment-processing kit17 and incised ochre nodules1 at Blombos Cave. At around the same time, humans at Pinnacle Point cave 13B and Klasies River Main site were collecting novel, non-utilitarian objects (non-food marine shells)8,11,18, possibly as a component of symbolic practices. At Diepkloof Rockshelter, the discarded fragments of decorated ostrich eggshell (OES) containers have been recovered in association with Howiesons-Poort-type artefacts, with the earliest undecorated OES dating to about 105ka3,19. Today, all of these archaeological sites are coastal, although this would have been different in the past20,21. However, even during the most extreme glacial periods of the past 120thousand years, the coast was never more than about 100km away21. Stratified Late Pleistocene archaeological sites with good preservation and robust chronologies are rare in the interior of southern Africa, which has resulted in a strong bias towards coastal sites that marginalizes the role of inland populations.
|
|
|
Post by Admin on Apr 6, 2021 19:37:21 GMT
Ga-Mohana Hill North RockshelterGa-Mohana Hill is located in the southern Kalahari Basin, 12km north-west of Kuruman (South Africa) and 665km from the nearest modern coastline. Ga-Mohana Hill North Rockshelter (GHN), is the largest of two main shelters and several small overhangs that occur within the dolomitic Gamohaan Formation (dating to the Palaeoproterozoic era). We excavated three areas of the shelter (4.75m2 in total) and reached a maximum depth of 1.7m, which revealed a sequence of stratified Middle Stone Age and Later Stone Age deposits22. Below about 10cm of loose surface sediments, we found three horizontally bedded stratigraphic aggregates that we refer to as ‘dark brown gravelly silt’, ‘orange ashy silt’ and ‘dark brown silt and roofspall’ (DBSR). The slope and orienta-tion of the plotted finds (n=2,128) indicated that the artefacts were in near-primary context22. Single-grain optically stimulated luminescence (OSL) dating of quartz from each of the three stratigraphic aggregates provided in-sequence dates with 1σ errors of 14.8±0.8ka (dark brown gravelly silt), 30.9±1.8ka (orange ashy silt) and 105.3±3.7ka (DBSR) (weighted mean, n=3)22.Our focus in this Article is on the DBSR deposits from areaA, which are the deepest sediments excavated thus far. An additional OSL sample from about 1.6m below the surface in the DBSR gave a date of 105.6±6.7 ka (Extended Data Table1), which is consistent with the previous age estimates22 and results in a new weighted mean for the DBSR of 105.2±3.3ka.The assemblage of flaked stone artefacts from the DBSR is typical of a Middle Stone Age assemblage, and is characterized by blades, points and prepared cores (Extended Data Fig.2, Supplementary Data). These artefacts are manufactured from locally available chert (42%), tuff (28%) and banded ironstone (26%). We also recovered a large piece of red ochre that bears evidence of use in the form of two flat surfaces, one of which exhibits shallow parallel striations (Extended Data Fig.2), from the DBSR. 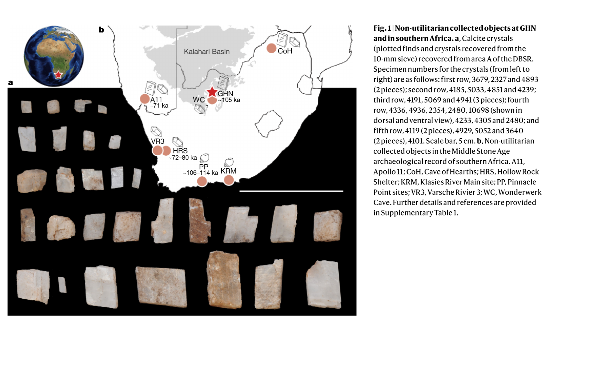 Calcite crystals We excavated 22unworked calcite crystals from the DBSR. The crystals are semi-translucent white rhombohedrons with regular, well-formed facets that range in maximum length from 0.8 to 3.2cm (Fig.1, Extended Data Table2). The crystals have not been introduced into the deposit from outside the shelter via natural processes; the DBSR artefacts lay flat within the horizontally bedded sediments, and they exhibit non-preferential orientations. The artefacts plot within the minimal slope wash zone of a Benn diagram22, which summarizes clast slope and orientation data for known disturbances on a ternary plot23,24. Diagenetic calcium minerals (such as gypsum) form in archaeological sediments as microcrystal-line aggregates that disrupt bone preservation25,26, but these are unlike the calcite crystals from the DBSR (which are macrocrystalline and do not disrupt bone preservation) (Supplementary Discussion). The calcite crystals in the DBSR do not originate from the shelter walls or ceiling. Although bladed calcite crystals do occur naturally as veins in the Gamohaan Formation27, they are too small (<0.5cm in maximum dimension) and irregular in form to be the source of the archaeological crystals (Extended Data Fig.3). Well-formed, white semi-translucent crystals do not occur naturally in the shelter walls or ceiling, or within the areas of Ga-Mohana Hill that we have intensively surveyed. A potential source for the calcite crystals is a low-lying dolomitic hill that is located 2.5km southeast of GHN, where we observed large rhombohedron calcite crystal formations as well as abundant quartz crystals (Extended Data Fig.4); this means that there is a local source for these crystals, although their natural occurrence is rare. None of the calcite crystals from the DBSR (nor indeed any from GHN) shows any signs of deliberate work-ing. It is unlikely that these crystals were transported to GHN to serve as raw material for stone tool production because calcite is soft (Mohs hardness of 3) and does not fracture conchoidally. The crystals have a limited distribution: the majority were recovered from two quadrants (0.5m2) and over a vertical distribution of less than 15cm (Extended Data Fig.5), which suggests that they were deposited as a discrete cache. The crystals are similar in size to crystals that we recovered from the over-lying Middle Stone Age and Later Stone Age stratigraphic aggregates (χ2=0.38, P=0.83, degrees of freedom=2). We therefore argue that the calcite crystals found in the DBSR represent a small cache of deliberately collected non-utilitarian objects.Crystals have been linked to spiritual beliefs and ritual for many time periods across the world, including Stone Age southern Africa28,29. Crystals are known from Holocene30–32 and Pleistocene30,33–38 contexts in southern Africa, but—to our knowledge—an assemblage of crystals has not previously been described from deposits that are robustly dated to over 80ka (Supplementary Discussion, Supplementary Table1). The collecting of non-utilitarian objects at GHN at around 105ka is thus coeval with the comparable practice of collecting non-food marine shells along the south coast of southern Africa8,11,18.
|
|
|
Post by Admin on Apr 7, 2021 6:03:43 GMT
Human-collected OES We recovered 42OES fragments from the DBSR. The pieces that com-prise the OES assemblage are fragmented, and the average maximum fragment length is 11.3mm (range of 5.3 to 25.7mm). Several lines of evidence provide support for an anthropogenic origin of the OES. First, the fragments occur within a well-preserved rockshelter context and are in direct association with many other traces of human activity (Extended Data Fig.5). Second, the OES fragments show evidence of having been burned. Over 80% (n=34) of the OES fragments display red colouration (Fig.2, Supplementary Data), which reflects exposure to temperatures of 300–350 °C39. Third, humans were the primary agents of accumulation for the faunal assemblage at GHN and there is no evidence for the presence of hyenas or other animals that consume ostrich eggs. The identifiable fraction of zooarchaeological material from the DBSR (n=467) is dominated by remains of ungulates and tortoises (Table1, Supplementary Data). 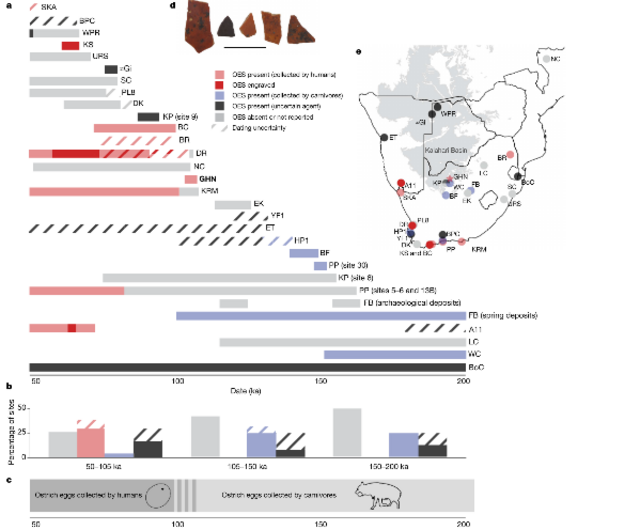 Fig. 2 | Distribution of OES across southern African archaeological sites dated to 50–200ka. This figure indicates all known southern Africa archaeological sites dated to between 50 and 200ka with preserved faunal remains. a, Date estimate ranges of archaeological sites, indicating the presence or absence of OES and the probable agent of its accumulation. Hashed lines indicate dating uncertainty owing to differing interpretations, contextual complexities or other factors. Dark grey indicates that the agent of accumulation has not confidently been determined. Further details and references are provided in Supplementary Table2. b, Frequency histogram of dated sites by time period. c, Interpretation of the timing of a shift in OES use by humans at around 105ka. d, Sample of OES fragments from the DBSR. Plotted find numbers (from left to right): 4942, 5040, 4917, 4265 and 4182. Scale bar, 2cm. e, Location of sites in a. BC, Blombos Cave; BF, Bundu Farm; BoC, Border Cave; BPC, Boomplaas Cave; BR, Bushman Rockshelter; DK, Die Kelders; DR, Diepkloof Rockshelter; EK, Erfkroon; ET, Erb Tanks; FB, Florisbad; HP1, Hoedjiespunt 1; KP, Kathu Pan (sites 6 and 9); KS, Klipdrift Shelter; LC, Lincoln Cave; NC, Ngalue Cave; PL8, Putslaagte 8; PP, Pinnacle Point (sites 30, 5–6, 13B);SC, Sibudu Cave; SKA, Spitzkloof A Rockshelter; URS, Umhlatuzana Rockshelter; WPR, White Paintings Rockshelter; YF1, Ysterfontein 1. Taphonomic analysis dem-onstrates a high frequency of anthropogenic percussion marks and cut marks (Table1), and most of the faunal specimens show evidence of moderate burning (Supplementary Data).Ethnographic observations suggest that OES can be used to create effective storage containers40, which represent a crucial innovation for early humans as they allow for the transport and caching41,42 of water and other resources. Evidence for OES containers are common in Later Stone Age assemblages43, and this technology has a long chronology at coastal sites in southern Africa that extends into the Middle Stone Age as far back as around 105ka19. To our knowledge, an assemblage of human-collected OES has not previously been reported from compara-ble contexts in the Kalahari (Fig.2, Supplementary Table2, Supplemen-tary Discussion): we show here that the appearance of human-collected OES at GHN is coeval with that at the coast.
|
|
|
Post by Admin on Apr 7, 2021 19:43:36 GMT
Palaeoenvironmental context Evidence from Ga-Mohana Hill provides key insights into the palaeoen-vironmental context of our findings. Tufa rim pools, barrages, cascades and breccia deposits are abundant at Ga-Mohana Hill, and are indicative of past shallow pools of standing water and flowing surface waters. Several phases of cascade formation occur on either side of GHN, both insitu against the dolomite and as exsitu collapsed blocks (Fig.3). Cores from an exsitu cascade yielded five uranium–thorium ages (Fig.3, Extended Data Table3) that gave a date range of 113.6 to 99.7ka. This semi-continuous tufa formation indicates abundant water flowing down the hillside. This timing agrees with other palaeoclimate records in the Kalahari Basin: a mega-lake high-stand in the Makgadikgadi Basin that has been dated using OSL to 104.6±3.1ka44 and sediments at Kathu Pan6 that are interpreted as relating to a marshy environment and that have been dated using OSL to 100±6ka45.Modern climate data informs on potential past drivers of climate change. Today, the Southern Oscillation index shows a significant posi-tive correlation with rainfall around Ga-Mohana Hill in November and May (Extended Data Fig.7), but not over the rest of the year. Indian Ocean sea surface temperature does not significantly correlate with rainfall around Ga-Mohana Hill, apart from a significant negative cor-relation with rainfall in the month of November (Extended Data Fig.7). Sea surface temperature reconstructions on the basis of marine core sediments (cores MD96-204846,47 and RC11-12048) for the Indian Ocean indicate a stronger dipole at around 110–100ka, which would have favoured convective uplift over South Africa. However, the magnitude of the dipole does not appear to be extreme enough to be the sole contributor to increased rainfall (Extended Data Fig.7, Supplementary Discussion). Thus, we propose that no single climate driver can explain increased rainfall but that instead a combination of warmer sea surface temperatures in the southwestern Indian Ocean and an intense negative Southern Oscillation index increased rainfall, which—together with the storage capacity in the dolomite bedrock—produced permanent water on the landscape (Supplementary Discussion). 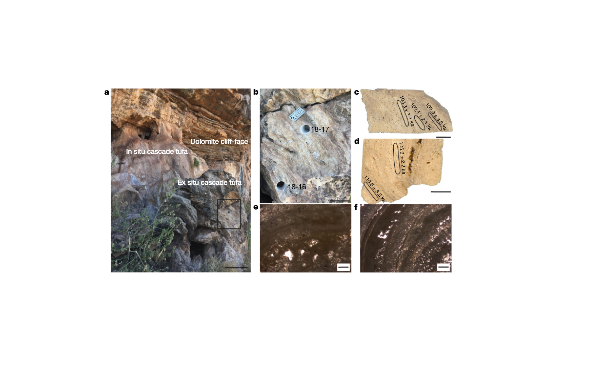 Fig. 3 | Dated cascade tufas. a, Field context of insitu cascade tufa built out from the dolomite cliff face, and collapsed exsitu cascade within close proximity. b, Close-up photograph of drill holes for samples 18-16 and 18-17 from the exsitu cascade. c, d, Pieces of cut and polished core samples (18-17 (c) and 18-16 (d)); areas outlined in black indicate subsampled layers and the associated dates. e, f, Thin-section images of cascade samples taken using a polarizing light microscope under plane-polarized light, showing layers of dense aphanitic micrite, mixed micrite and microspar, and void spaces. Scale bars, 0.5mm (e, f), 1cm (c, d), 10cm (b), 50cm (a). Discussion Single-origin coastal models for the evolution of behaviourally modern H.sapiens assume that human populations in the interior of Africa had little or no role in the emergence of key cultural inno-vations. However, our excavation of well-dated Middle Stone Age deposits at GHN in the southern Kalahari Basin has yielded evidence that conflicts with these coastal models, which—given the rarity of dated Late Pleistocene sites far from the coast—have always been problematic. Our findings at GHN push back the evidence for collect-ing non-utilitarian objects and OES in the Kalahari to about 105ka, which is coincident with the evidence from coastal contexts. The newly uncovered record of behavioural innovation at GHN is coeval with a period of increased rainfall in the Kalahari, and contradicts the longstanding notion of an empty and arid interior. A greater avail-ability of water is correlated with increased primary productivity and greater population densities49, which in turn may have had an influence on the origin and spread of innovative behaviours50. The archaeological, dating and palaeoenvironmental methods used here set the foundation for further investigations in the Kalahari and other understudied regions.Evidence from GHN calls into question the reliance on coastal resources for the emergence of human behaviours that are regarded as uniquely modern. Instead, these behaviours appear to be shared across diverse and distant environments and could reflect techno-logical convergence or rapid social transmission via interconnected populations spread throughout Africa. Thus, our results add support for a polycentric origin for the emergence of H.sapiens7,51–54 that is not limited to coastal environments and that includes the southern Kalahari Basin.
|
|
|
Post by Admin on Apr 10, 2021 4:38:16 GMT
The primitive brain of early Homo Science 09 Apr 2021: Vol. 372, Issue 6538, pp. 165-171 DOI: 10.1126/science.aaz0032 Human brains are substantially larger than those of our closest living relatives, the great apes, and also bear evidence of important structural reorganization, notably in cortical association areas related to higher cognitive functions, such as toolmaking and language capabilities (1, 2). Clarifying when these structural innovations appeared during human evolution remains a major challenge (3, 4). Brains do not fossilize, and so the only direct physical evidence for the actual course of brain evolution comes from natural or virtual fillings of fossil hominin brain cases, so-called endocasts, which show a complex but spatially reliable pattern of imprints representing cerebral gyri and sulci, as well as vascular structures surrounding the brain (5). The endocranial region that holds key information about frontal lobe reorganization and possible language capabilities is Broca’s cap (BC), a bulge on the lateral fronto-orbital surface of endocasts (Fig. 1). BC is present on both human and great ape endocasts and often assumes similar morphologies (6, 7). However, the underlying brain areas are not the same across groups (7, 8) (Fig. 1). In great apes, BC largely comprises Brodmann area 44, and its inferior border is formed by the fronto-orbital sulcus (fo) (Fig. 1A). In humans, BC largely comprises Brodmann areas 45 and 47, and its inferior delimitation tends to coincide with the lateral orbital sulcus (9) (Fig. 1B). Inferring brain reorganization from the morphology of the BC region of fossil endocasts thus remains ambiguous. The BC of Australopithecus endocasts is typically interpreted as being delimited inferiorly by fo, indicating a great ape–like organization of the underlying brain areas (7). However, endocranial evidence from Australopithecus africanus and Australopithecus sediba has been interpreted as showing incipient reorganization of the frontal lobe (10, 11). This proposition has been challenged on various grounds (12, 13), such that basic questions about the timing and mode of the evolutionary transition from great ape–like to humanlike frontal lobe organization remain largely unanswered.  Fig. 1 Topographical relationships between neurocranial and brain structures on endocasts of great apes and humans. Cranial sutures are indicated in blue, cerebral sulci in red, and brain regions in other colors. (A) In great apes, the precentral sulcus (pc) crosses the coronal suture (CO), such that its inferior portion (pci) is located anterior to CO. The lunate sulcus (L) marks the anterior border of the primary visual cortex (Brodmann area 17). (B) In humans, evolutionary expansion of the inferior prefrontal cortex (IPF) resulted in a shift of the pci toward the posterior side of CO (red arrowheads). Concomitant expansion of the parietal bone resulted in anterior shift (blue arrowheads) of the apical portion of CO. Expansion of the posterior parietal cortex (PP) resulted in fragmentation and eventual disappearance of L. Numbers indicate Brodmann areas. Neurocranial structures: BC, Broca’s cap; CO, coronal suture; LA, lambdoid suture. Cerebral sulci: c, central; fi, inferior frontal; fs, superior frontal; fo, fronto-orbital; ip, intraparietal; lo, lateral orbital; pc (pcs/pci), precentral (superior/inferior); pt, postcentral; ts, superior temporal; s, Sylvian (lateral); L, lunate; R/Rʹ, ascending/horizontal rami of anterior lateral sulcus. Vascular structures: SS, sigmoid sinus; TS, transverse sinus. Figure was redrawn from (34, 35). Uncertainties also exist regarding the evolutionary reorganization of the parieto-occipital cortex. All great ape brains exhibit a lunate sulcus (L), which marks the anterior boundary of the primary visual cortex (Brodmann area 17) (Fig. 1A), whereas human brains are characterized by complete loss of L (14), reflecting an expanded parieto-occipital cortex (13, 15) (Fig. 1B). L rarely leaves endocranial imprints (7, 16, 17), such that, in fossil hominin endocasts, absence of evidence of L is not evidence of absence, and it remains unclear when during human evolution this cortical area started expanding (3, 4, 7, 9, 18–20). Traditionally, it has been assumed that a derived, humanlike organization of the frontal and parieto-occipital lobes (see legend of Fig. 1) characterizes the genus Homo from its beginnings (21–23). However, testing this hypothesis explicitly on fossil endocasts has proven difficult. The African fossil record extends back to the origins of Homo at around 2.8 million years ago (Ma) (24), but critical endocranial evidence is only preserved from ~1.8 Ma onward and represented by single finds dispersed in space and geological time (Table 1). This makes it difficult to identify diachronic trends in brain evolution against a background of interindividual variation in endocranial morphology. Homo erectus from Southeast Asia, on the other hand, is represented by numerous well-preserved neurocrania from a geographically restricted area, but with younger geological dates (25, 26) (Table 1). The fossil hominins from the early Pleistocene site of Dmanisi, dated to 1.85 to 1.77 Ma (27, 28), play a key role in assessing brain reorganization in early Homo and its potential importance for hominin dispersals from Africa. Dmanisi has yielded five exceptionally well-preserved crania representing individuals from adolescence to old age, which likely represent natural variation in a paleodeme of early Homo (27, 29–32). Also, the site preserves a rich record of faunal remains and Mode I (Oldowan) lithic artifacts co-occurring in taphonomic context, which permits inferences on tool use and site-specific behaviors of the Dmanisi hominins and, more broadly, on subsistence strategies, social organization, and cognitive capabilities of early Homo (28, 30, 31). Here, we track key changes in brain organization of early Homo from ~1.8 Ma onward by analyzing the endocranial morphology of the five Dmanisi specimens and reevaluating an extended comparative sample of African and Southeast Asian fossil endocasts (33) (Table 1). To identify primitive versus derived aspects of frontal lobe organization in fossil endocasts, we use the highly distinct cranio-cerebral topographies of humans and great apes as a frame of reference (Fig. 1) (34, 35). A characteristic signal of inferior frontal lobe expansion is the posterior shift of the inferior precentral sulcus (pci) relative to the coronal suture (CO) (35) (Fig. 1). Both structures are typically well represented on fossil endocasts, such that their topographical relationships reliably indicate frontal lobe reorganization (supplementary method M3). In addition, we use geometric morphometric methods to identify which endocranial and brain regions underwent differential expansion during frontal lobe reorganization (supplementary methods M4 and M5).
|
|