|
Post by Admin on Dec 24, 2021 19:24:54 GMT
The Late Neolithic/Early Chalcolithic Genetic Structure in Anatolia, Northern Levant, and Caucasian Lowlands So far, our knowledge of the gene pool of Neolithic Anatolia has been limited to individuals from Barcın and Menteşe in Western Anatolia (abbreviated here as “Barcın_N”) (Mathieson et al., 2015), Boncuklu from the Konya Plain in Central Anatolia (Feldman et al., 2019, Kılınç et al., 2016), and Tepecik-Çiftlik in Southern Anatolia (Kılınç et al., 2016). These individuals date from the 9th to the 7th millennium BCE and are succeeded by LN/EC individuals of this study. To overview the genetic structure in this Near Eastern region from the Neolithic to the Bronze Age, we first performed principal-component analysis (PCA) (Patterson et al., 2006, Price et al., 2006) of present-day West Eurasians populations and projected ancient individuals onto the top PCs (Figure 3A). Overall, LN/EC individuals are scattered along PC2 between Barcın_N and ancient individuals from Iran/Caucasus (Figure 3B). TellKurdu_EC are slightly shifted along PC1 toward Neolithic and Chalcolithic Levantine individuals. Büyükkaya_EC is positioned further away from any Neolithic Anatolian reported to date and toward the direction of Neolithic and Chalcolithic Iranian individuals. Caucasus_lowlands_LN (two individuals from Polutepe and Mentesh Tepe) are positioned upward along PC2, between Büyükkaya_EC and Chalcolithic Iran.  Figure 3. Principal-Component Analysis Principal-component analysis (PCA) was computed on the Human Origins (HO) SNP panel data of present-day West Eurasian populations (gray symbols) and ancient individuals were projected on them. (A) PC1 and PC2 for ancient individuals from the present study and selected from previous publications. (B) PC1 and PC2 for individuals by archaeological time or geographic sector (a–d) with some of the important findings annotated. To formally test the qualitative differences observed in PCA, we compared the genetic affinity of LN/EC groups to earlier populations in Western Eurasia by computing f4-statistics (Patterson et al., 2012) of the form f4(Mbuti, p2; p3, X) (Figure 4). The statistic deviates from zero if a pair of Anatolian/Levantine/Caucasian groups (p3 and X) differ from each other in their genetic affinities to Epipaleolithic and Neolithic populations (p2). We observe that Büyükkaya_EC and Caucasus_lowlands_LN differ from Barcın_N by sharing more alleles with Caucasus hunter-gatherers (CHG; Satsurblia and Kotias Klde caves) and Iran_N (Ganj Dareh site in Zagros mountains) than with Barcın_N (+2.2 to +5.5 SE), while sharing less alleles with hunter-gatherers from Western Europe (WHG) (≤−4.3 SE), Early European Farmers (EEF) (≤−3.6 SE), the Epipaleolithic Pınarbaşı individual from Anatolia (≤−6.8 SE), and with the Neolithic/Epipaleolithic Levant (−1.3 to −9.4 SE). By summarizing the f4-statistics using qpAdm (Haak et al., 2015), we can adequately model both Büyükkaya_EC and Caucasus_lowlands_LN as a two-way mixture of Barcın_N and Iran_N as source populations (p ≥ 0.083; 24%–31% from Iran_N; Figure 4). Tepecik_N, which occupies an intermediate position between Barcın_N and Büyükkaya_EC in the PCA, also fits the same model (p = 0.975; 22% from Iran_N). By replacing Iran_N with CHG, we still obtain a good model fit for Büyükkaya_EC (p ≥ 0.825; 24% from CHG), but not for Caucasus_lowlands_LN (p = 0.0001).  Figure 4. Genetic Affinity of Late Neolithic/Early Chalcolithic Populations with Early Holocene Populations from Iran, Caucasus, and Levant Measured with f4-Statistics and Quantified with qpAdm f4-statitstic tests whether either p3 or X has excess affinity with p2 and becomes negative or positive accordingly, as shown in the simplified tree. SE for f4-statitstics are estimated by 5 cM block jackknifing and values that do not deviate from 0 in the ±3 SE are represented in gray color. All three groups have more affinity with Iran compared to Barcın_N (green bar), and TellKurdu_EC has the more Levantine affinity compared to all (purple bar). These affinities are reflected in the inferred qpAdm models on the right. Ancestry proportions are plotted with ±1 SE. Consistent with their positions on the PCA plot, TellKurdu_EC does not fall on this cline of mixed Barcin_N-Iran_N ancestries but shows extra affinity with ancient Levantine populations. Accordingly, f4-statistics of the form f4(Mbuti, Levant_N; X, TellKurdu_EC) ≥3.3 SE, show that TellKurdu_EC has more affinity with the pre-pottery Neolithic Levantines (“Levant_N”) than with any other Neolithic-Early Chalcolithic (“N-EC”) Anatolian population including an almost 1,000-year younger individual from the same area (TellKurdu_MC). When compared to Barcın_N, TellKurdu_EC has significantly (<−4 SE) less affinity with Mesolithic hunter-gatherers from Western, Eastern, and Southeastern Europe (WHG, EHG, and Iron_Gates, respectively). The admixture model with Barcın_N+Iran_N/CHG used above is not supported for TellKurdu_EC (p < 1.47 × 10−5). Instead, we can successfully model TellKurdu_EC as a three-way mixture of Barcın_N, Iran_N (or CHG), and Levant_N (p = 0.298; 15.5% ± 3.7% from Iran_N and 36.6% ± 7.1% from Levant_N; Figure 4).
|
|
|
Post by Admin on Dec 24, 2021 20:37:52 GMT
Neolithic Admixture and a Common Genetic Profile of Chalcolithic and Bronze Age Groups In contrast to the LN/EC individuals, LC-LBA individuals form a dense cloud in the West Eurasian PCA, roughly falling mid-way along the LN-EC cline that is delimited by ancient groups from Iran, the Caucasus, the Levant and Western Anatolia. We hypothesize that LC-LBA groups from Central/North and Eastern Anatolia may have descended from this older genetic structure and therefore share the same ancestry profile. Consistent with PCA, outgroup-f3 and f4-statistics suggest a common genetic profile of the LC-LBA groups that is similar to that of the LN-EC cline. First, outgroup f3(Mbuti; LC-LBA, Test), which measures the average shared genetic drift between LC-LBA and Test from their common outgroup Mbuti (Patterson et al., 2012), reached highest values when Test were Neolithic and Chalcolithic populations from Europe, Anatolia, and Northern Levant, such as Barcın_N, TellKurdu_EC, and Büyükkaya_EC (Table S4). Second, using Barcın_N and additionally TellKurdu_EC as local baselines, we computed f4(Mbuti, Test; Barcın_N/TellKurdu_EC, X) to characterize the difference between Barcın_N or TellKurdu_EC and the LC-LBA groups (X) with respect to a set of ancient Test populations from West Eurasia (Table S5). Iran Neolithic and/or CHG consistently show excess affinity to LC-LBA when compared to TellKurdu_EC and Barcın_N. The Chalcolithic and Bronze Age populations from Iran (Iran_C from the Seh Gabi site) and the Caucasus (Allentoft et al., 2015, Lazaridis et al., 2016, Wang et al., 2019)—temporally closer to LC-LBA and located between Iran_N/CHG and LC-LBA in the PCA—also occasionally only share more alleles with some of the LC-LBA groups when compared to Barcın. We further explored the temporal aspect of the shared admixed profile of LC-LBA groups by estimating their admixture dates using the recently developed method, DATES (M. Chintalapati, N. Patterson, N. Alex, and P. Moorjani, personal communication) (STAR Methods). As we previously described, the LN-EC cline as varying proportions of Barcın_N and Iran_N/CHG ancestries, we selected both as source populations. However, given the small sample size of both Iran_N and CHG and the large number of missing SNPs in Iran_N, we also considered modern Caucasians (Armenians, Georgians, Azerbaijanis, Abkhazians, and Ingushians) as proxies of the second source population. We focused on the three Late Chalcolithic groups with sufficiently large sample size and who are the earliest in time among the LC-LBA groups: ÇamlıbelTarlası_LC (n = 9), İkiztepe_LC (n = 11), and Arslantepe_LC (n = 17). Taking individual estimates from all these individuals together (“Anatolia_LC”), we obtain a robust admixture date estimate of 105 ± 19 generations ago when we use Barcın_N and modern Caucasians as proxies of the source gene pools (Figure 5A). Using a generation time of 28 years (Moorjani et al., 2016), this estimate equates to an admixture event ∼3,000 years before the time of the LC-LBA individuals, corresponding to ∼6500 years BCE (Figure 5B). We observe similar but noisier estimates from individual groups ÇamlıbelTarlası_LC, İkiztepe_LC, and Arslantepe_LC. Admixture dates estimated by two alternative methods, ALDER and rolloffp (STAR Methods), overall matched our estimates with DATES (Figure S2).  Figure 5. Dating of Admixture in Anatolian and Caucasian Populations from the Late Neolithic to the Early Bronze Age (A) Decay of ancestry covariance estimated by DATES for Anatolia_EC (Büyükkaya_EC) and Caucasus_lowlands_LN grouped together and the three Late Chalcolithic populations Arslantepe_LC, ÇamlıbelTarslası_LC, and İkiztepe grouped as “Anatolia_LC.” (B) Conversion of admixture dates into calendar dates (upper part of plot) after including both the age range for each population calculated from direct 14C dates (lower part of plot) and the ±1 SE from DATES. Average population and admixture dates are shown with black dot. Average admixture date for the three populations with grouped individuals (bold letters) is 6500 years BCE. Admixture dates for individual populations from Anatolian_LC span a wider time range.  Figure S2. Summary of Admixture Dates Estimated with Alder and Rolloffp, Related to STAR Methods (Test of Recent Admixture) (A) Alder admixture dates on all pair combinations from 27 Anatolia_LC individuals. Pairs include individuals from the Arslantepe_LC and ÇamlıbelTarlası_LC groups. Estimates for which computation of SE failed are not plotted. The average admixture date from the 241 independent tests (red dot) is very close to the estimation from DATES on Anatolia_LC (~100 generations). (B) rolloffp estimates of admixture dates overlap with DATES within ±1 SE. Encouraged by these results, we extended the analysis to other ancient populations that are on the Early Chalcolithic cline, including Caucasus_lowlands_LN and Büyükkaya_EC, published Early Bronze Age (EBA) individuals from the Caucasus (cluster a) (Wang et al., 2019, Lazaridis et al., 2016) (see Table S3 for group labels), as well as Iran_C. For the Caucasus EBA individuals (“Caucasus_a_EBA”), dated to ∼3,100 years BCE, which is similar to the Late Chalcolithic Anatolian individuals, we obtain a similar admixture date of 121 ± 35 generations. Importantly, the earlier two Caucasus_lowlands_LN and the one Büyükkaya_EC individuals yielded more recent admixture dates of 34 ± 15 generations before the age of the individuals (∼5600 years BCE) (Figure 5A). The converted calendar date of ∼6500 years BCE matches the timing of the admixture event estimated from the Late Chalcolithic individuals (Figure 5B).
|
|
|
Post by Admin on Dec 25, 2021 3:50:08 GMT
Admixture Modeling of the Chalcolithic and Bronze Age Groups Although we showed that it requires both Barcın_N and Iran_N-related ancestries to explain the ancestry composition of the LC-LBA groups, alternative combinations of ancient populations, which may be temporally and spatially more proximal to the LC-LBA groups, may also provide equally fitting models. To obtain a plausible admixture model that more likely reflects the true demographic history, it is crucial to precisely distinguish between closely related candidate source populations. We used qpAdm to model all LC-LBA groups as a mixture of two sources, one related to the Neolithic Anatolian ancestry and the other related to Iran and the Caucasus populations (Tables S6 and S7). For the former, we used three Neolithic or Early Chalcolithic groups (Barcın_N/TellKurdu_EC/Büyükkaya_EC). For the latter, we used Iran_N and CHG, as well as more recent Chalcolithic and Bronze Age populations from the same region (Allentoft et al., 2015, Lazaridis et al., 2016, Wang et al., 2019). Although the admixture signal in LC-LBA is older than these later populations, we nonetheless used them as a proxy because they might represent a gene pool that is not yet sampled but had contributed to LC-LBA individuals. We find that Barcın_N+Iran_N adequately explain many LC-LBA groups, but it fails for Alalakh_MLBA, Ebla_EMBA, Arslantepe_LC, Barcın_C, and Caucasus_lowlands_LC (p < 0.05). Iran_N-related contribution varies from 21% ± 9% to 38% ± 6% (Figure 6A). The alternative model of Barcın_N+CHG provides slightly higher estimates for CHG-related contribution from 27% ± 13% to 41% ± 7%, although most groups (8/12) cannot be modeled with CHG. For the Chalcolithic and Bronze Age groups, Iran_C provides similar results with Iran_N but with a higher contribution (34%–53%; p ≥ 0.05 for 8/12 groups). Iran_C itself can be modeled as a mixture of Iran_N and Barcın_N (p = 0.365; 37% ± 3% from Barcın_N), which corresponds well with the modeling results for LC-LBA. In contrast, those from the Caucasus, specifically the Eneolithic to Bronze Age (_En/BA) groups, mostly fail to fit LC-LBA.  Figure 6. qpAdm Modeling of Ancient Anatolian, Northern Levantine, and Southern Caucasian Populations from the Present and Previously Published (∗) Studies (A) When we model the ancient populations as Western Anatolia (Barcın_N) and Zagros (Iran_N), LN/EC populations fall on a spatial gradient of Iran_N-like ancestry which is attenuated in the subsequent LC-LBA populations. Vertical bars represent ±1 SE estimated by 5 cM jackknifing. (B) LC-LBA can be modeled as the geographically proximal source Büyükkaya_EC from Central Anatolia, with contribution from Iran_N that ranges from 0% in GonrdürleHöyük_EBA from Western Anatolia and ~30% in Caucasus_lowlands_LC. In order to model Ebla_EMBA and Alalakh_MLBA with Büyükkaya_EC and Iran_N extra contribution from a source like Levant_N is necessary. See also Figure S3 and Tables S4, S5, S6, S7, and S8. We repeated our admixture modeling by replacing Barcın_N with TellKurdu_EC. Models with TellKurdu_EC in general provide a good fit to the LC-LBA groups, although we caution that it may be an artifact of reduced statistical power for detecting discrepancies between the model and the actual target groups, due to the much smaller sample size of TellKurdu_EC (n = 5) compared to Barcın_N (n = 22). While models with ancient Iranian populations fail for multiple LC-LBA groups (p < 0.05 for 5/12 with Iran_N and for 3/12 with Iran_C), TellKurdu_EC+CHG can model all LC-LBA groups with varying level of CHG contribution ranging from 13% ± 19% to 40% ± 9%, except for Barcın_C. Replacing CHG with a later Caucasus group (“G.Caucasus_a_En”) provides the same pattern with a higher Caucasus-related contribution (40%–67%; p ≥ 0.05, also with the exception of Barcın_C). When we repeated the analysis after adding Barcın_N to the outgroup set, most results remained similar. However, two LC-LBA groups from the same region with TellKurdu_EC (i.e., Ebla_EMBA and Alalakh_MLBA) became deviant from the model (p < 0.03), suggesting that TellKurdu_EC may not be a good local proxy in a simple two-way admixture model. Therefore, it seems to hold that ancient Iranian groups overall serve as a better proxy than the Caucasus groups, although higher resolution data are necessary to compare them further. Büyükkaya_EC is the earliest individual in our dataset with a genetic profile similar to the LC-LBA groups within Anatolia. Therefore, we also tested a scenario in which the later LC-LBA groups had descended from the same gene pool without further external contribution. f4(Mbuti,X;Büyükkaya_EC,LC-LBA) suggests that Büyükkaya_EC shares more alleles with the European/Anatolian Farmers (e.g., Boncucklu, LBK, Barcın_N) than LC-LBA groups do (Table S5). Likewise, most LC-LBA groups cannot be modeled in qpAdm as a sister group with Büyükkaya_EC when Barcın_N is included in the outgroups (p < 0.05 for 1-way model for 11/12). Most LC-LBA groups are adequately modeled by adding the second ancestry of ancient Iran/Caucasus populations, while Alalakh_MLBA and Ebla_EMBA require a substantial contribution from ancient southern Levantine populations (Figure 6B). Overall, the inference of the same admixture date drawn from both Late Neolithic and Late Chalcolithic populations combined with the qpAdm analyses suggest that the LC-LBA populations also derived from the Neolithic genetic cline but were substantially more homogenized than their predecessors (Figure 6A). Although the ancient groups from Iran are a better proxy for the eastern source than those from the Caucasus, we caution a naive literal interpretation of our results, as yet unsampled proxies from within Mesopotamia may represent true historical sources of this Iran/Caucasus-related ancestry.
|
|
|
Post by Admin on Dec 25, 2021 20:21:42 GMT
Genetic Turnover in the Bronze Age Northern Levant The Northern Levant, represented by the sites Tell Kurdu, Ebla, and Alalakh, showcases the most noticeable genetic turnover among our four time transects. Within two millennia after the last Middle Chalcolithic Tell Kurdu individual (TellKurdu_MC), the genetic profile of the populations in and around the Amuq valley (Alalakh_MLBA and Ebla_EMBA) changed to largely resemble contemporaneous Anatolians. However, the qpAdm modeling with Büyükkaya_EC suggests that Alalakh_MLBA and Ebla_EMBA are still distinct from the other Anatolian groups with respect to their connection to the ancient Southern Levant. Their distinction is also captured by f4-statistics whereby Ebla_EMBA and Alalakh_MLBA differ from the other LC-LBA groups with respect to their relation with older populations such as Barcin_N and Caucasian groups (Figure S3). In addition, Barcin_N/TellKurdu_EC and/or ancient Caucasian groups cannot successfully model Ebla_EMBA and Alalakh_MLBA in qpAdm (Tables S6 and S7), suggesting that these sources do not represent good proxies of their true ancestries. Alternative models with the predecessor TellKurdu_EC as a baseline ancestry and the geographically close Arslantepe_LC as a potential proximal source did not improve the fit either (p ≤ 1.3 × 10−5; Table S8). However, admixture models become adequate by adding a southern Levantine population as the third source, with significant Levantine contributions: e.g., 27%–34% TellKurdu_EC + 36%–38% G.Caucasus_a_En + 28%–38% Levant_EBA (p ≥ 0.492).  Figure S3. Genetic Differences among Analyzed Chalcolithic and Bronze Age Groups, Related to Figure 6 Heatmap of f4-statistics of the form f4(Mbuti, p2; p3,p4), where p3 and p4 are all possible pairs of LC-LBA groups from the present study and published contemporaneous (∗), and p2 a selection of ancient populations from West Eurasia. f4-statistics that do not deviate significantly from 0 (i.e., |Z-score| ≤ 3) are represented with gray-colored tiles. Significant f4-statistics are colored in red and blue scale according to the direction of allele sharing. f4-statistics estimated on less than 50,000 SNPs are not plotted resulting in some missing tiles from the heatmaps. Consistent with the extra gene flow after the time of TellKurdu_EC, we obtain younger admixture dates in Alalakh_MLBA than the other LC-LBA groups when we use either Anatolian or Caucasian gene pools as sources: 78 ± 27 generations (3880 ± 746 years BCE) with Anatolia_LC and 44 ± 8 (3060 ± 224 years BCE) with Caucasus_a_EBA. No exponential decay could be fitted when we used either Anatolia_LC or Caucasus_a_EBA as one source and Levant_C as a second. Evidence for Individual Mobility in Alalakh All genetic analyses of Alalakh_MLBA were conducted at the exclusion of one female (ALA019) because of her outlier position in the PCA (Figure 3B). Discovered at the bottom of a well, the archaeological and anthropological context suggest that the skeletal remains of this woman, C14-dated to 1568–1511 cal BCE (AMS; 2-sigma), represent an irregular burial with evidence of several healed skeletal traumata (Figure 7; STAR Methods). We have ruled out the possibility of modern-day contamination based on the characteristic aDNA damage profile, low mitochondrial contamination and reproduction of the PCA coordinates after discarding all sequence reads without damage (STAR Methods). In the Eurasian PCA (Figure S4), ALA019 falls genetically closer to Chalcolithic and Bronze Age individuals from ancient Iran and Turan (present-day area of Iran, Turkmenistan, Uzbekistan, and Afghanistan) (Narasimhan et al., 2019). These populations represent a west-east genetic cline with varying proportions of ancestries related to Barcın_N, Iran_N and WSHG (hunter gatherers from Western Siberia). We confirmed the genetic affinity of ALA019 observed in the PCA with an outgroup-f3 test (Figure 7A). Other ancient populations from the Caucasus and the Western steppe also produced high affinity but f4(Mbuti, X; Turanx, ALA019) suggest that ALA019 differs from other Turan individuals by occasionally sharing more or less alleles with either Iran_N or WSHG (Figure 7B), which agrees with the presence of a genetic cline in this area. In the absence of ancient genomes from nearby regions such as Southern Mesopotamia, the most likely ancestral origin of this individual was somewhere in Eastern Iran or Central Asia.  Figure 7. Individual ALA019 from Alalakh Has High Genetic Affinity to the Ancient Contemporaneous Populations from Eastern Iran and Turan (Central Asia) (A) Shared genetic drift measured with outgroup f3-statistics between ALA019 and ancient Eurasian (diamonds) and worldwide modern (circles) populations. The highest five values of the test are produced by populations from Turan which are labeled. (B) Symmetry f4-statistics show variable affinity to Iran_N and WSHG among Turanx and ALA019. Horizontal bars represent ±3 SE estimated by 5 cM jackknifing. Photo of “Well Lady” (ALA019): Murat Akar, Alalakh Excavations Archive.
|
|
|
Post by Admin on Dec 25, 2021 21:20:14 GMT
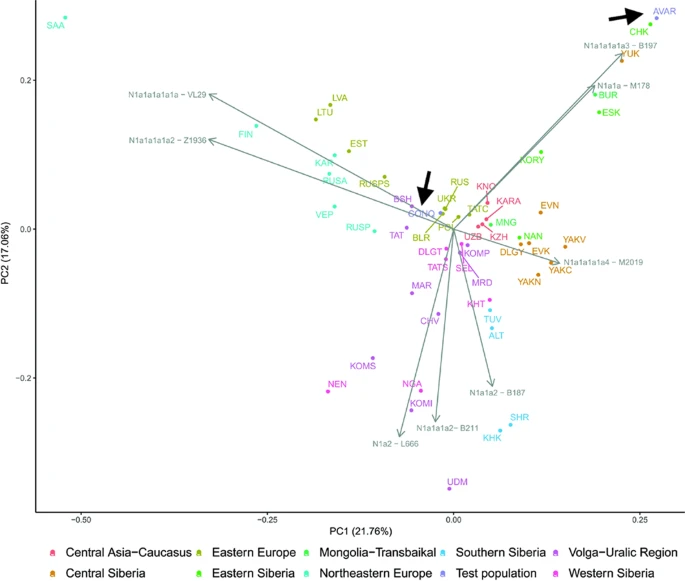 Figure S4. Eurasian PCA with Neolithic to Bronze Age Individuals from Iran and Turan and the Genetic Outlier Individual from Alalakh (ALA019), Related to Figure 7 Scatter plot of PC1 and PC2 from PCA computed on modern-day Eurasian populations (gray points) shows that the Alalakh_MLBA_outlier (ALA019) is genetically closer to individuals from Chalcolithic and Bronze Age Iran/Turan. Colored labels and points refer to ancient populations and black labels to modern-day populations. Discussion Genetic Homogenization across Anatolia and the Southern Caucasus prior to the Bronze Age Our study covers time transects of ∼4,000 years of Syro-Anatolian and ∼2,000 years of Southern Caucasian history, both starting from the 6th millennium BCE. In addition, our admixture date estimates allow us to infer a millennium further back in time to the Neolithic period. We describe a Late Neolithic/Early Chalcolithic (6th millennium BCE) genetic cline stretching from Western Anatolia (i.e., area around the Sea of Marmara) to the lowlands of the Southern Caucasus that was formed by an admixture process that started at the beginning of Late Neolithic (∼6500 years BCE). The eastern end of this cline extends beyond the Zagros mountains with minute proportions of Anatolian (i.e., Western Anatolian-like) ancestry reaching as far as Chalcolithic and Bronze Age Central Asia (Narasimhan et al., 2019). To the south, Anatolian ancestry is present in the Southern Levantine Neolithic populations (Lazaridis et al., 2016), and to the north, in the Chalcolithic and Bronze Age populations from the Caucasus (mainly mountainous area) (Allentoft et al., 2015, Lazaridis et al., 2016, Wang et al., 2019), most likely as a result of the Late Neolithic admixture. Evidence for genetic homogenization across larger geographic distances also comes from the uniparentally inherited Y chromosome lineages (Table S9). We observe the most common male lineages J1a, J2a, J2b, and G2a in all spatiotemporal groups of the region. Alongside the less frequent lineages H2 and T1a, these all form part of the genetic legacy that dates to the Neolithic or was already present in the region during the Upper Paleolithic (Wang et al., 2019, Lazaridis et al., 2016, Jones et al., 2015, Feldman et al., 2019, Broushaki et al., 2016). A few notable exceptions provide rather anecdotal but nonetheless important evidence for long distance mobility and extended Y-haplogroup diversity. For example, individual ART038 carries Y-haplotype R1b-V1636 (R1b1a2), which is a rare clade related to other early R1b-lineages, such as R1b-V88 that was found in low frequency in Neolithic Europe (e.g., Haak et al., 2015) and R1b-Z2103—the main Y-lineage that is associated with the spread of “steppe ancestry” across West Eurasia during the early Bronze Age. However, R1b-V1636 and R1b-Z2103 lineages split long before (∼17 kya) and therefore there is no direct evidence for an early incursion from the Pontic steppe during the main era of Arslantepe. Lineage L2-L595 found in ALA084 (Alalakh) has previously been reported in one individual from Chalcolithic Northern Iran (Narasimhan et al., 2019) and in three males from the Late Maykop phase in the North Caucasus (Wang et al., 2019). These three share ancestry from the common Anatolian/Iranian ancestry cline described here, which indicates a widespread distribution that also reached the southern margins of the steppe zone north of the Caucasus mountain range. Dating the formation of the west-to-east cline during the 7th millennium BCE enables the contextualization of these genetic signals observed on both autosomal and uniparental markers with archaeological evidence of human mobility and changes in socio-cultural practices. The time around 6500–6400 years BCE marks a significant junction in the Anatolian Neolithic because it saw a sudden and massive expansion of sedentary communities into areas that had previously supported no or very few food-producing communities before (Brami, 2015, Düring, 2013). Subsequently, in the Southern Caucasus, the abrupt appearance of a Neolithic lifestyle and the introduction of exogenous domesticated animal and plant species ca. 6000 BCE suggests some type of interaction with, and eventually intrusion of Neolithic populations from the neighboring regions, among which Southeastern Anatolia—along with Zagros and the Caspian belt—could be one of the most suitable candidates (Chataigner et al., 2014). Related to these events, the genetic structure of domesticated caprine populations within the Near East began to break down, and by the Chalcolithic period, goat herds across the region were found to harbor ancestries both from eastern and western Neolithic populations (Daly et al., 2018, Kadowaki et al., 2017). Although the exact timing of this admixture is not known, the parallel between human and livestock genetic histories suggests that livestock moved not only through trade networks but also together with people, as well as their material culture, ideas, and practices. This is indicated, for instance, by the circular Neolithic architecture of the Southern Caucasus (Baudouin, 2019, Lyonnet et al., 2016), which is reminiscent of the Halaf traditions, that were developing during the early 6th millennium in North Mesopotamia and along the Anatolian stretches of the Tigris and Euphrates river valleys. In the subsequent millennia and until the Late Bronze Age, genetic continuity persisted in North-Central and Eastern Anatolia, which is supported by the genetic similarity of these later populations and the absence of new ancestry sources after the Neolithic period. This contradicts prior hypotheses for population change based on archaeological evidence of intense cultural interactions during this period. For example, the site of İkiztepe on the Turkish Black Sea Coast contains a material culture with strong Balkan affinities, and this has been argued to signify direct contact with populations across the Black Sea (e.g., Thissen, 1993), but these contacts do not seem to be accompanied by gene flow. The site of Arslantepe provides another representative example. At the beginning of EBA, archaeological evidence at the site strongly suggests the presence of a disruptive socio-political conflict that led to the occupation of Arslantepe by pastoral populations with a connection to the Caucasus. In PCA and f4-statistics, two individuals dating to this period show excess affinity with populations from the Caucasus and the Pontic steppe compared to their peers, while later Arslantepe_EBA individuals do not share this Caucasian affinity (Table S10). This implies that the postulated demic interaction must have been transient and of small scale, although the small sample size from Arslantepe_EBA (n = 4) may not be sufficient to detect it. Subtle gene flow is consistent with recent findings from the site, suggesting that the EBA pastoralists occupying the site were more likely well-established local groups moving around the mountains rather than intrusive groups from the Caucasus (Frangipane, 2014). The genetic landscape of Arslantepe also has important implications with respect to the interactions with the Mesopotamian world. Archaeological evidence indicates that in the 4th millennium BCE Mesopotamian populations established colonies in Southeastern Anatolia and Northern Syria (e.g., Habuba Kabira, Jebel Aruda, Hacinebi) during a period referred to as the Uruk Expansion (Algaze, 2005). However, the Uruk expansion was also a very complex and deep process of socio-cultural transformation that reoriented the economic, political, and cultural interests of indigenous elites toward Southern Mesopotamia. Artifacts in Arslantepe reflect this complexity, and the genetic continuity shown here supports the notion that indigenous populations adopted these broader Uruk features and ideas without the transmission of genes. Population and Territorial State Dynamics in the Northern Levant In contrast to the rest of Anatolia, the Northern Levant stands out as a region of the Near East with traceable post-Neolithic changes in the genetic structure. We found that the gene pools at Ebla and Alalakh can only be explained by more complex models that require additional contributions both from the Caucasus and Southern Levant. The inclusion of a source related to the Caucasus in our proposed model raises the question whether this turnover could be linked to the expansion of the Transcaucasian Kura-Araxes material culture to the Levant. This expansion is recorded in the region of the Northern Levant ca. 2800 BCE and could be associated with the movement/migration of people from Eastern Anatolia and the Southern Caucasian highlands (Greenberg and Palumbi, 2015, Greenberg et al., 2014). However, our results do not support this scenario for a number of reasons: (1) we do not find any substantial increase of Caucasus-related ancestry in the populations of the primary expansion area of Kura-Araxes (e.g., Eastern Anatolia), (2) populations from the highlands of the Southern Caucasus—including individuals from a Kura-Araxes context (“L.Caucasus_EBA”)—as secondary source populations also fail, and (3) so do models with Arslantepe from Eastern Anatolia, a population located mid-way along the proposed expansion route from the Southern Caucasus to the Northern Levant. Consequently, these interpretative caveats call for consideration of alternative historical scenarios, including scenarios of multiple gene-flow events that could have taken place in the intervening two millennia between the Tell Kurdu population and those of Bronze Age Ebla and Alalakh. However, written sources, archaeological, and paleoclimatic evidence suggest that a narrower time period—the end of the EBA—had been very critical with respect to political tensions and population mobility. It was during this period, for example, that Ebla was destroyed twice and re-established at the beginning of MBA. There are extensive textual references from the end of the EBA through the LBA referring to groups of people arriving into the area of the Amuq Valley. Although these groups were named, likely based on designations (e.g., Amorites, Hurrians), the formative context of their (cultural) identity and their geographic origins remain debated. One recent hypothesis (Weiss, 2014, Weiss, 2017, Akar and Kara, 2020) associates the arrival of these groups with climate-forced population movement during the “4.2k BP event,” a Mega Drought that led to the abandonment of the entire Khabur river valley in Northern Mesopotamia and the search of nearby habitable areas. Taking the above into consideration, we suggest that the ancestries we inferred for Alalakh and Ebla might best describe the genetic make-up of the EBA populations of unsampled Northern Mesopotamia. During the following MBA and LBA, we find no evidence of genetic disruption, even though shifts in territorial control dynamics between kingdoms/empires affected Ebla’s and Alalakh’s socio-cultural development (see STAR Methods). Nevertheless, the case of the Alalakh individual with likely Central Asian origin is a finding that can be interpreted within the context of nascent internationalism of the Middle and Late Bronze Age Eastern Mediterranean societies. It calls for future research on the various societal features of this phenomenon and how these are reflected on the individual life histories. Conclusions Overall, our large-scale genomic analysis reveals two major genetic events. First, during the Late Neolithic, gene pools across Anatolia and the Southern Caucasus mixed, resulting in an admixture cline. Second, during the Early Bronze Age, Northern Levant populations experienced gene flow in a process that likely involved yet to-be-sampled neighboring populations from Mesopotamia. Even though we could detect subtle and transient gene flow in Arslantepe, we acknowledge that disentangling questions related to local-scale population dynamics within the homogeneous Chalcolithic and Bronze Age Anatolian gene pool might be beyond the resolution of current analytical tools. Furthermore, while our sampling expands in number and geographic range on previous studies, the critical area of Mesopotamia remains unsampled; thus, although our picture of the genetic landscape of the Near East is highly suggestive, it remains incomplete. Nevertheless, the cumulative genetic dataset of Anatolia, the Southern Caucasus, and the Northern Levant between the Early and Late Bronze Age indicates that, following the genetic events of the Late Neolithic and Early Bronze Age, there was no intrusion of genetically distinct populations in this region. This conclusion is of great importance with respect to our understanding of the formation of complex Bronze Age socio-political entities.
|
|